Latest Features
What is A Ventilator And Why Is It So Important?
Forget rebreathers. With the pandemic still spreading, ventilators have arguably become one of the most essential pieces of life-support kit on the planet. We asked anesthesiologist and director of Karst Underwater Research (KUR) Andy Pitkin to brief us on these life-saving devices. Take a deep breath.
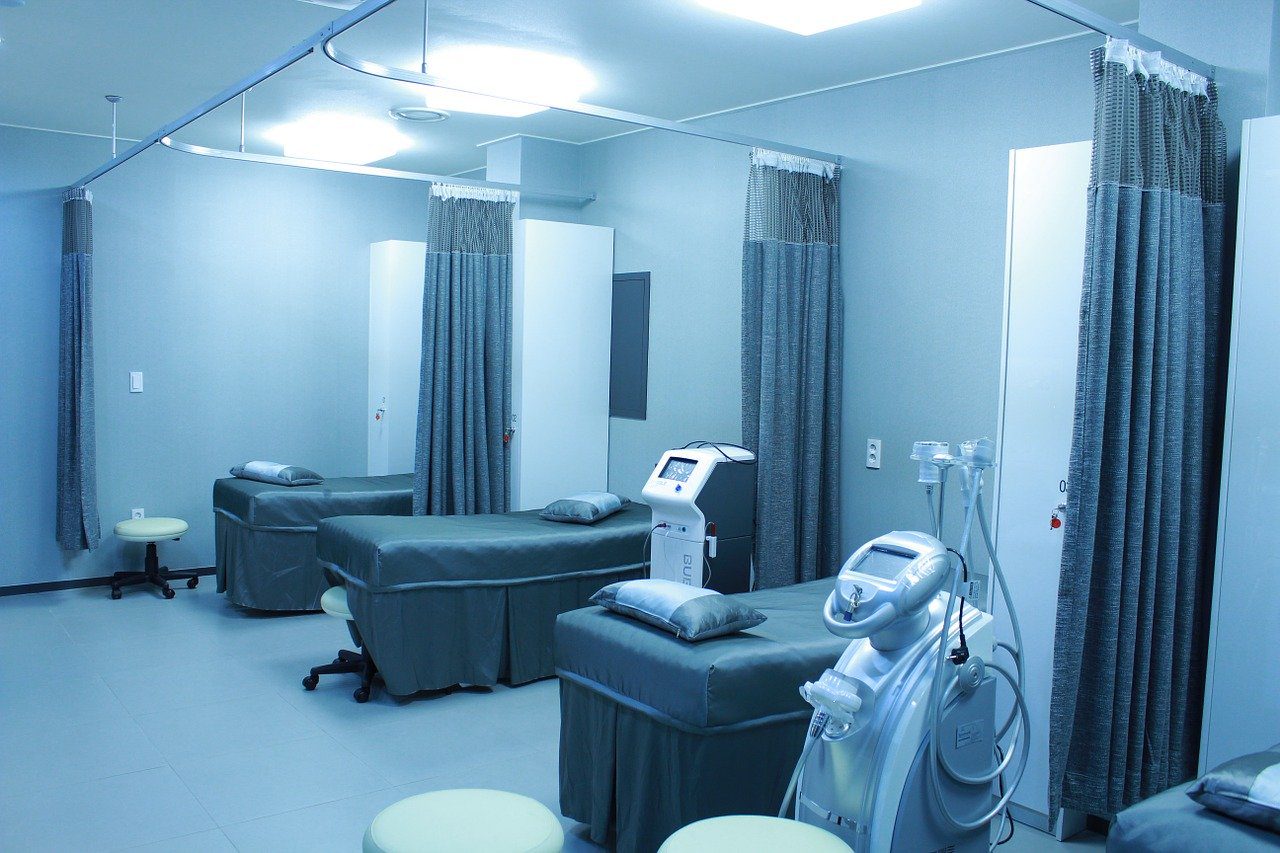
by Andrew Pitkin MBBS MRCP FRCA
Header photo by Sungmin Cho from Pixabay
The COVID-19 pandemic has highlighted one piece of medical equipment above all: the ventilator. What are these life-support devices, and how do they work?
Until the 20th century, with a few exceptions, any patient unable to breathe for themselves was doomed to die. The large polio outbreaks that swept through the world in the 1930s and 40s prompted the design and manufacture of the first reliable means of respiratory support, the iron lung. Properly termed a negative pressure ventilator, and commonly referred to as a ‘tank’ ventilator, the iron lung and some smaller derivatives enclosing just the chest (‘cuirasse’ ventilators) were developed by Drinker1 and Emerson into the only equipment able to keep patients alive when their respiratory muscles had been paralyzed by the poliovirus. Well-funded hospitals in the USA were able to set up large wards full of iron lungs (Fig 1), but it was across the Atlantic, in the northern European country of Denmark, that the modern intensive care unit with its ubiquitous positive-pressure ventilators was born.
In the mid-1950s, Copenhagen, Denmark’s capital city, was ravaged by a severe polio epidemic that completely overwhelmed its handful of iron lungs and forced the physicians there to adopt methods borrowed from the operating room just to keep people alive. In desperation, the director of the infectious diseases hospital, the Blegsdamhospitalet, asked his anesthesiologist colleague Dr Bjorn Ibsen for help. Under Ibsen’s direction, patients received tracheostomies and intermittent positive-pressure ventilation using a rebreathing system called a Waters circuit (Fig 2).
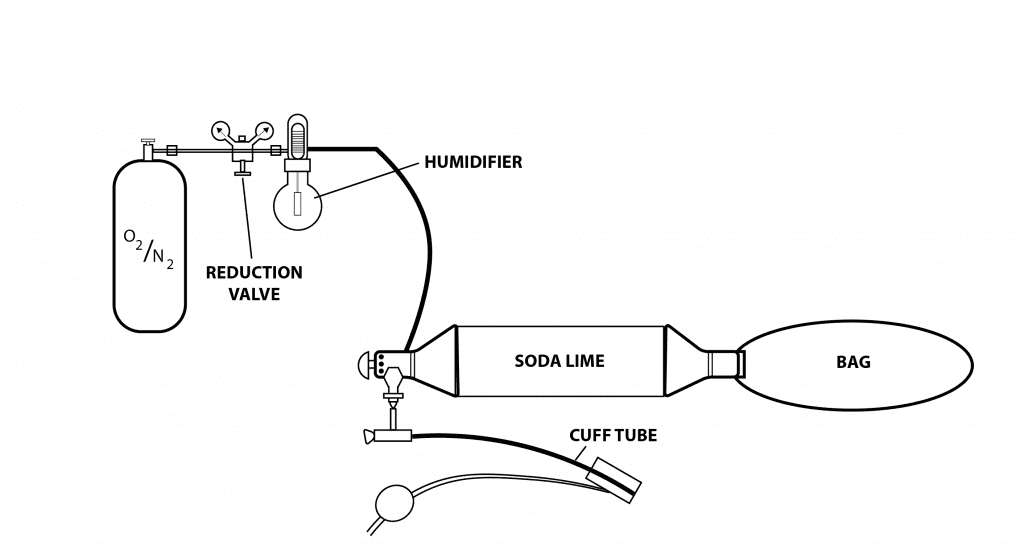
Rebreather divers will easily recognize this as a pendulum rebreather system, but in this case the gas moved in and out of the patient’s lungs not through their own efforts, but by a medical student squeezing and releasing the bag (counterlung) 20 to 25 times per minute, 24 hours a day, for periods of up to several months. In the absence of enough mechanical ventilators, hundreds of medical students risked exposure to the much-feared polio virus to keep these patients, many of them children, alive (Fig 3). The success of positive-pressure ventilation in dramatically reducing mortality in Denmark rapidly spread throughout the world and the modern intensive care unit was born.
Human muscle power was soon replaced with mechanical power using either motorized bellows or a pressure-regulated gas source controlled by an automatic valve (like pressing the purge valve on a regulator’s second stage). The first classification by Mapleson2 divided ventilators into flow generators and pressure generators; both could be further subclassified into time-cycled, pressure-cycled and volume-cycled units, depending on what parameter the machine used to switch from inspiration (breathing in) to expiration (breathing out) and vice versa. An example of a flow generator is a typical bellows ventilator such as the Oxford Penlon unit (Fig 4), still used in some hyperbaric chambers because of its mechanical simplicity, and many anesthesia machines in operating rooms have similar ventilators built in.
Modern Ventilators
With advances in microelectronics in the 1970s it became feasible to integrate gas control valves with flow and pressure sensors to allow a single ventilator to work in a flow-generating mode as well as a pressure-generating mode, and many other modes became possible that could not be realized with a simple mechanical ventilator. Units such as the Siemens Servo 900 (Fig 5) and the Puritan Bennett 7200 were in the vanguard of this approach.
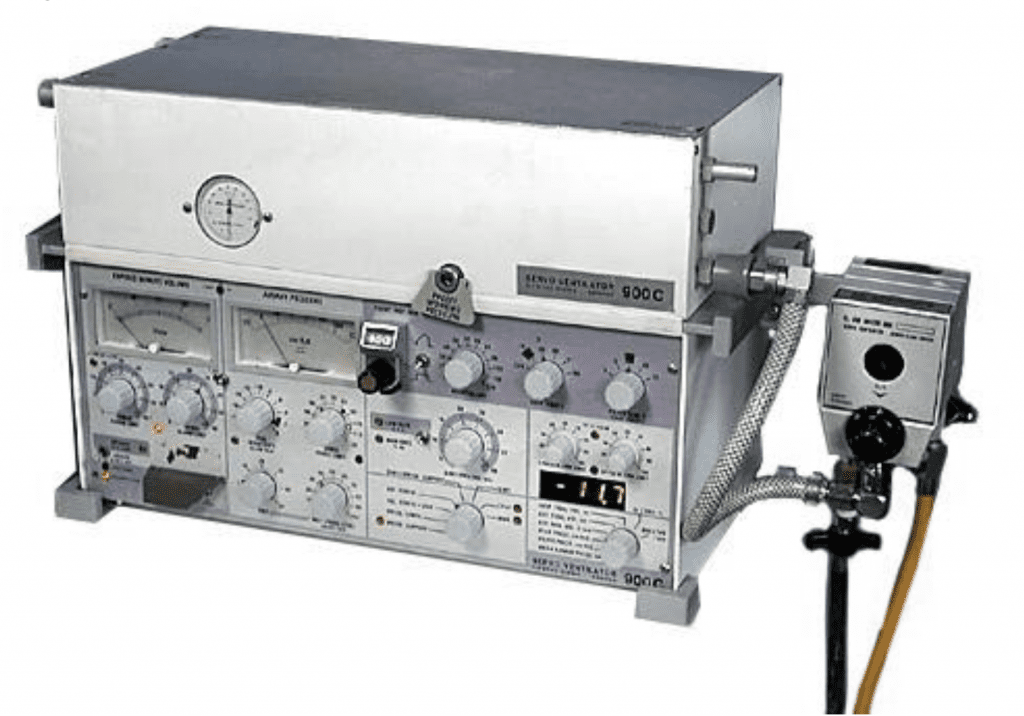
Modern intensive care ventilators have evolved into complex computer-controlled systems that operate with a degree of precision, flexibility and sophistication that would astonish Ibsen and his contemporaries (Fig 6). In the rare cases that adequate oxygenation and/or carbon dioxide clearance cannot be achieved with conventional ventilators, highly specialized options such as high frequency oscillatory ventilation (HFOV) and extracorporeal membrane oxygenation (ECMO) also exist.
A patient with respiratory failure must be intubated with a breathing (endotracheal) tube in the windpipe (trachea) which connects to the ventilator, and to tolerate this they often need sedation and occasionally chemical paralysis to prevent them from “fighting” the ventilator, although most modern ventilators have useful support modes that synchronize the ventilator with the patient’s own respiratory efforts. An alternative to the endotracheal tube placed through the mouth, especially useful in the longer term, is to place a tube in the trachea through the front of the neck (tracheostomy), and this approach undoubtedly contributed to Ibsen’s success in Copenhagen as many polio patients had paralysis of the muscles of their throat and voicebox (larynx) and so were unable to protect their lungs from contamination by coughing. Both endotracheal tubes and tracheostomies bypass the normal humidification that occurs in the upper airways so humidification of the gas supplied to the patient is important to prevent drying out of the lining of the airways.
Current medical practice is to set tidal volumes at 6-8 ml/kg (ideal body weight) and adjust respiratory rate to achieve the desired carbon dioxide (CO2) clearance. Almost all modern ventilators can be time-cycled (most common), pressure- and volume-cycled. Patients are generally most comfortable when the partial pressure of CO2 in their blood (PaCO2) is just below normal but with severe respiratory failure it is frequently better to allow it to rise above normal as long as adequate oxygenation can be maintained rather than subjecting diseased lungs to a higher tidal volume or respiratory rate – this is called permissive hypercapnia. Oxygenation is largely determined by the fraction of inspired oxygen (FiO2) and mean (average) airway pressure, the latter being influenced by a number of ventilator settings such as inspiratory/expiratory time ratio, positive end-expiratory pressure (PEEP) as well as patient factors such as lung compliance. For complex reasons it is common for other organ systems such as the heart and kidneys to fail in patients with respiratory failure, so for them to survive it requires a skilled team of doctors, nurses and other staff to monitor and intervene when problems occur.
Bjorn Ibsen went on to establish the first real intensive care unit, which he called an ‘observation unit’, at the municipal hospital of Copenhagen where he was appointed chief of the anesthesiology department in 1954. He widened its scope to include any patient who needed respiratory or cardiovascular support for any reason, such as trauma, post-operative complications, sepsis and so on. One of the pioneering features was a multidisciplinary team approach that has stood the test of time and is still used today. Another was the idea that no matter what the original disease, the same principles of organ support apply. These ideas are so universally accepted now that it is hard for us to conceive of a time when they were not.
Ventilators and the Covid-19 Virus
At the time of writing this, ventilators look like they could soon be in short supply and they cost tens of thousands of dollars. Why are they so complex and expensive? It is relatively easy to build a system of valves and sensors and an electronic control system that will function, but quite another to do so to the (justifiably) high standards of design quality, reliability, safety, ease of use and infection control that modern medicine demands. Getting any medical device approved by the US Food and Drug Administration (FDA) is very expensive and time-consuming, and similar regulatory processes exist in Europe and the rest of the world. Nevertheless, some would argue that medical device costs (like medications) are out of control, but essentially the price of sophisticated medical technology is a balance between its development costs and the price that the market will support.
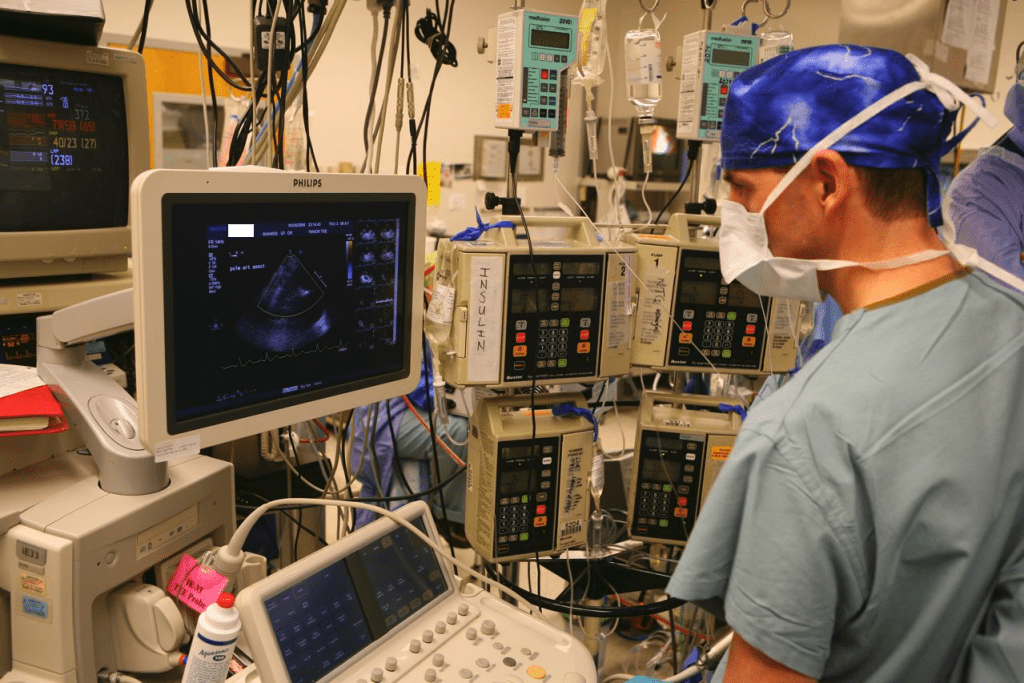
The COVID-19 pandemic has spawned a number of projects to tackle the projected shortage of ventilators with homebuilt systems. Usefully, the Medicines and Healthcare products Regulatory Agency (MHRA) in the United Kingdom has published a set of specifications for a Rapidly Manufactured Ventilator System (RMVS), a minimum standard that such a ventilator has to meet to be useful, based on expert opinion3. These efforts are to be applauded, but use of such a ventilator would be close to a last resort, after all the ventilators in anesthesia machines in every operating room were in use. What is more, it doesn’t address the issue that patients with severe COVID-19 disease often have multiple organ involvement and it is the highly-skilled and cohesive medical and nursing teams to apply the principles that Ibsen and others pioneered that are the real limiting factor.
References
- Drinker P, McKhann CF. The use of a new apparatus for prolonged administration of artificial respiration. JAMA 1929; 92: 1658-61.
- Mapleson WW. The effect of lung characteristics on the functioning of artificial ventilators. Anaesthesia 1962;17:300-14.
- https://www.gov.uk/government/publications/specification-for-ventilators-to-be-used-in-uk-hospitals-during-the-coronavirus-covid-19-outbreak accessed April 17th, 2020.
Dive Deeper
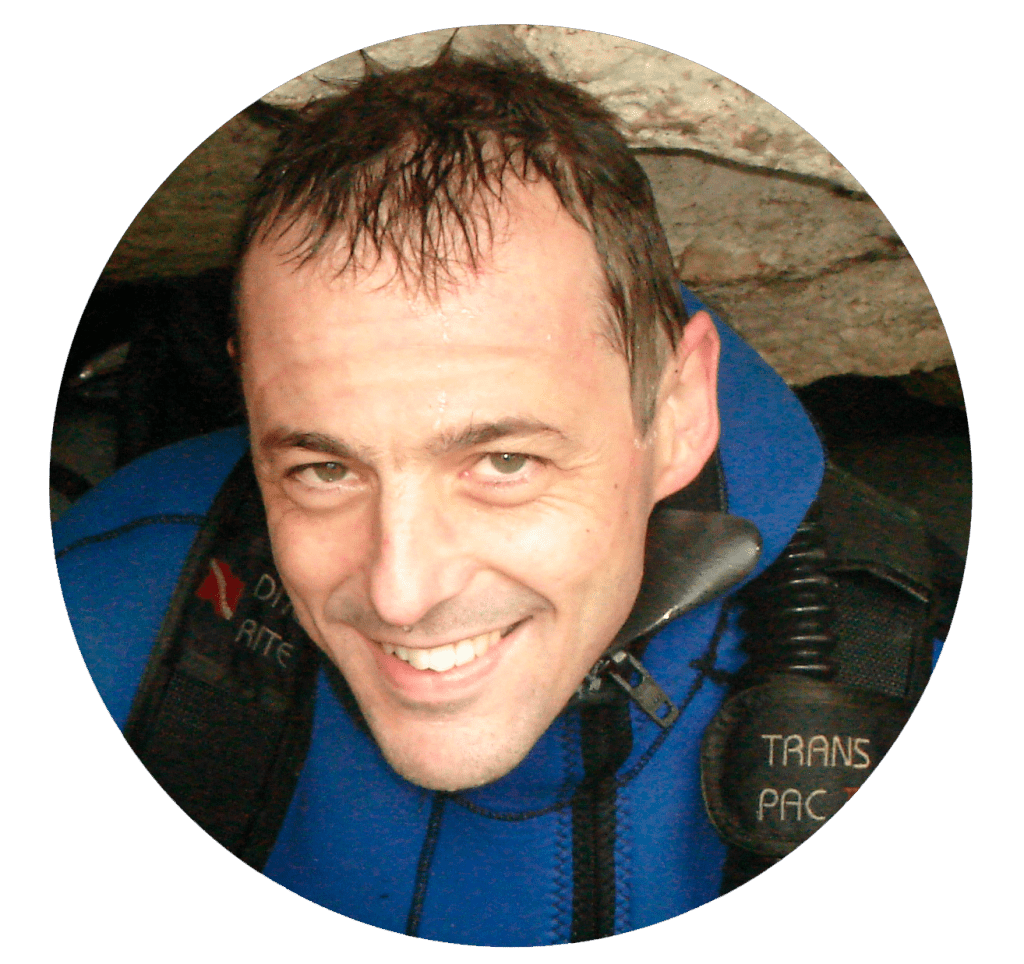
Andrew Pitkin learned to dive in 1992 in the cold murky waters of the United Kingdom and started cave and technical diving in 1994. His first exposure to exploration was in 1995 when he was one of a team of divers who were the first to reach the bottom of the Great Blue Hole of Belize at 408 fsw (123 msw). Subsequently he has been involved in numerous cave exploration projects in Belize, Mexico and Florida.
From 1996-2000 he was employed at the Royal Navy’s Institute of Naval Medicine, running a hyperbaric facility, treating decompression illness, participating in research into outcome after decompression illness, submarine escape and testing of new military underwater breathing systems. He is one of a handful of civilians to be trained by the Royal Navy as a diving medical officer.
He moved to Florida in 2007 and is currently on the faculty of the College of Medicine at the University of Florida in Gainesville. With Karst Underwater Research he has participated in numerous underwater cave exploration and filming projects. Like many explorers, he spends much of his spare time developing and building innovative equipment for exploration purposes.