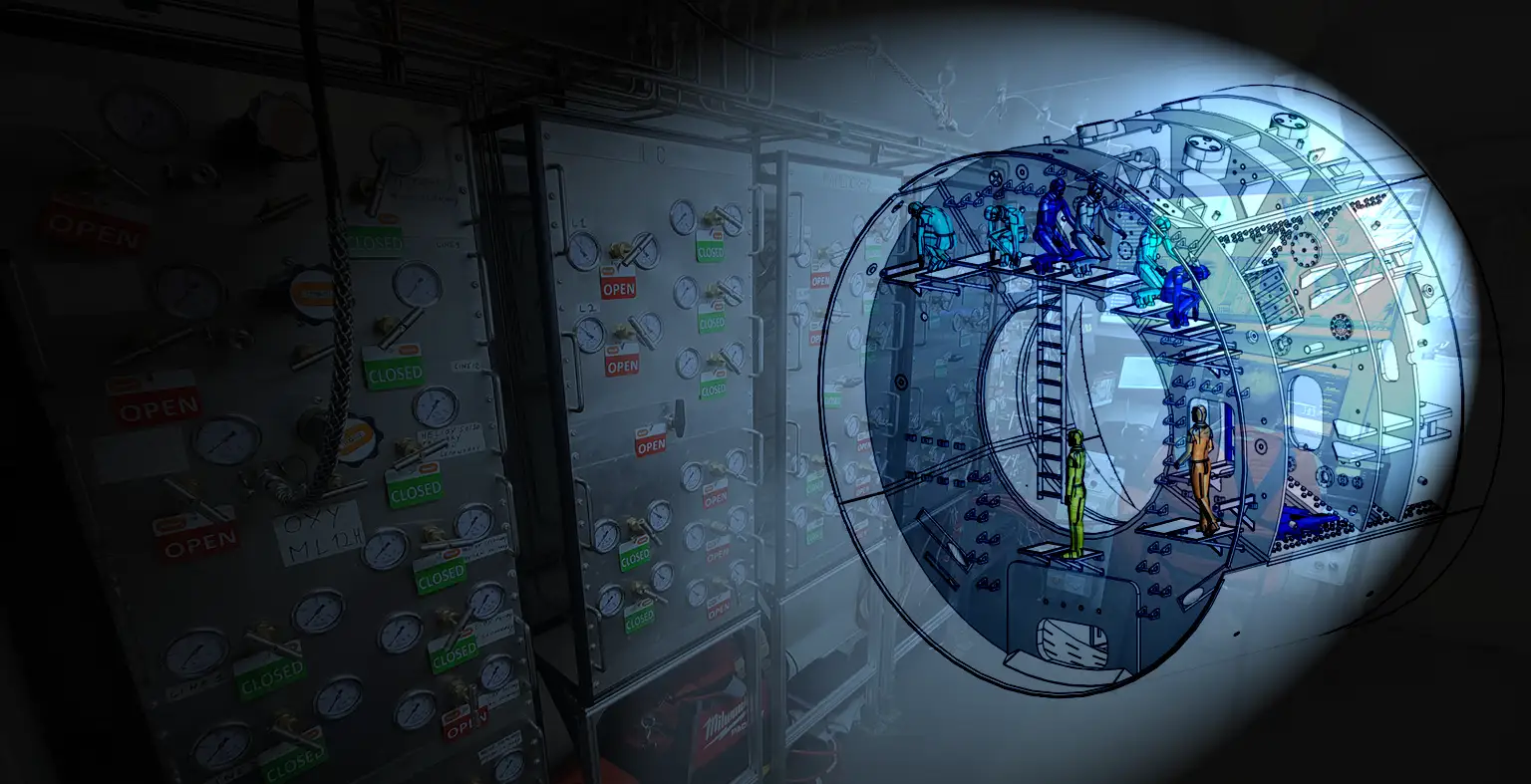
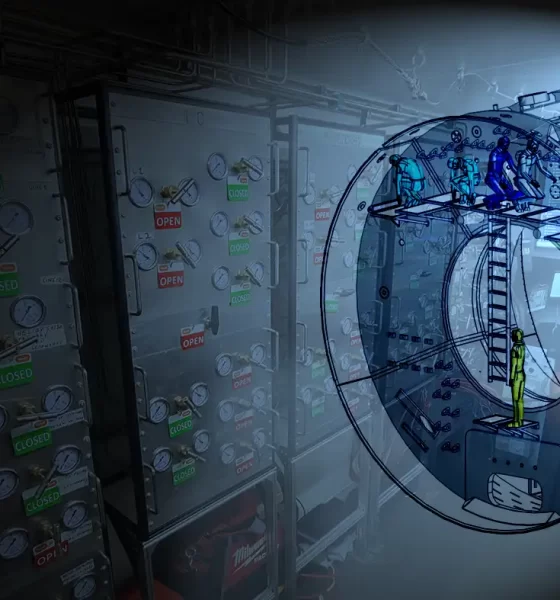
Diving Medicine
Deep Tunnel Workers Go Trimix
Deep tunnel boring workers are the modern equivalent of compressed air caisson workers—the poor souls who discovered the bends. Jump to modern day: During a job in Hong Kong, a tunneling contractor ran into a problem that required employees to work under pressure up to 5.5 bar (~atm) for hours. They turned to commercial decompression engineer and former tekkie JP Imbert to create special workplace tables. Imbert created novel tables with trimix that proved effective, enabling the humongous Tunnel Boring machine (TBM) to continue its $500k/day operation. Here Imbert, and cave instructor-cum-tunneling engineer Armin Sidali share their story.
By JP Imbert and Armin Sidali. Images courtesy of the authors unless noted. 3D models created by Armin Sidali.
“To Air is Human, To Trimix is Divine,” IANTD bumper sticker, early 1990s
Armin never smiles. His dress code is black on black. When he throws the ball, people run. He likes to say that his bad reputation is his best asset.
Armin and I are friends. We met when we were both International Association of Nitrox & Technical Divers (IANTD) instructors. At the time, he was getting good at cave diving. He is now managing hyperbaric interventions for a large tunnel project in Hong Kong. If asked how he went from cave to tunnel, I might say, “Because he is a dangerous perfectionist.”
One fateful day, Armin’s tunnel boring machine (TBM) was digging its way under the bay when it hit pink granite close to the shore. Pink does not mean soft. In fact, it is such hard rock that if you hammer it, you get a spark, not a chip of rock. As it struck the granite, the TBM started vibrating a warning, but the engineers kept pushing on the rams until they finally melted all the cutting tools. Not only that, but the ceiling soil collapsed and the front part of the TBM flooded with seawater. At the surface, the air escaping from the tunnel created a giant jacuzzi that made the evening news.
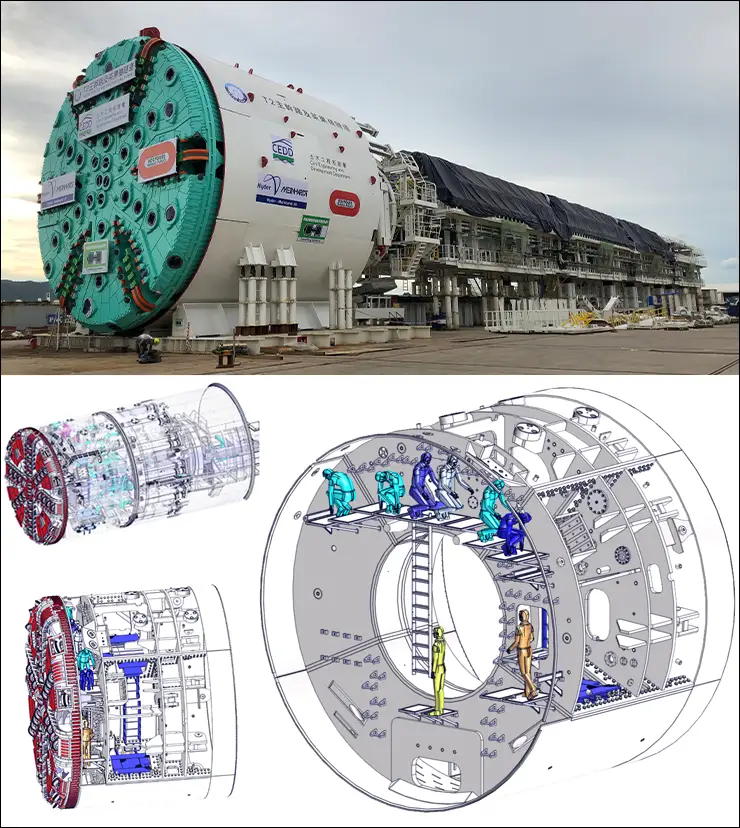
I am a diving engineer, working in the North Sea. The lives of diving engineers are getting boring today as the ROVs now get all the deep work. In the standard 0-200 msw range/0-650 ft, companies just keep doing what they have done before .
However, when I saw the video of the accident with the TBM, I figured Armin and I would soon be on stage. To put it in perspective, the TBM alone costs around half a million dollars a day to function, and it was stuck.
The standard way to solve such a problem is to freeze the ground—that is to order a tanker ship of liquid nitrogen and pump it into the soil until everything turned to ice—and then retrieve the TBM and repair what remained of it. That said, with Hong Kong’s tropical temperatures such a procedure could take as long as a year.
But Armin proposed a second option: diving into the flooded TBM!
Diving Into the TBM
Unlike cave diving, tunnelling fits into a specific regulatory and industrial category. Armin had started his project with a risk assessment of the situation: the professional equivalent of “What if?”
First, the equivalent depth ranges from 42-45 m/138-148 ft. The bottom time required would be 120 minutes at least in order to set tools and work efficiently. Therefore, I needed a table for 120 minutes at 45 m, which required efficient equipment and a precise approach.
Second, the medium would be water first and, after the front chamber had been sealed, hopefully compressed air next. Compressed air is for tires, and the workers would be masked and on mix. But, in case of leaks in the mask seal, the safety analysis suggested that it would be good to have a small partial pressure of nitrogen in the breathing mix to avoid a narcosis shock.
Third, the place was narrow, the work extreme, and (when dry) the temperature reached 40-45 °C/104-113 °F. Workers would soon become dehydrated. Moreover, the workers needed to repeat their intervention day after day for a month or two. During the planning process, I needed more than a “Jesus factor” in the selection of table depths and times; I wanted a documented safety margin for these difficult dive conditions and multi-day operations.
At the end of his risk analysis, Armin concluded that he needed specific trimix tables that did not exist.
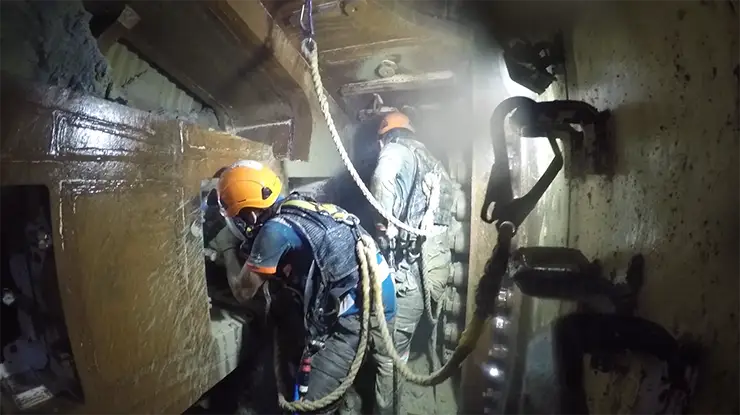
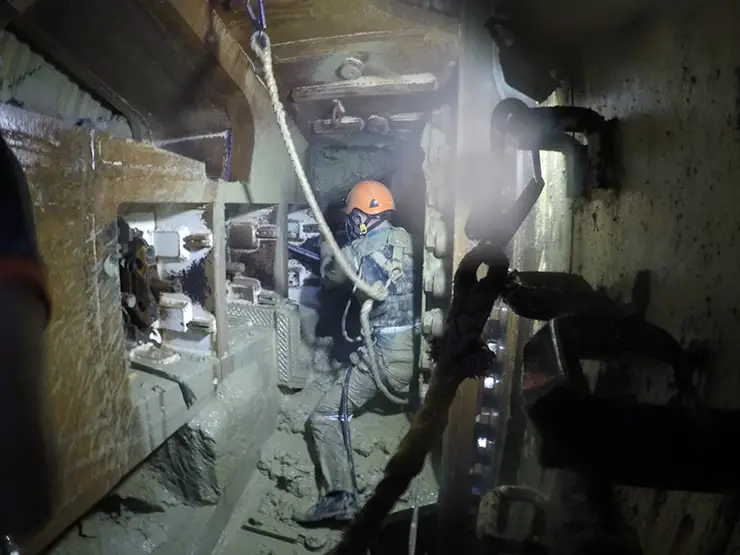
Armin took the project to the next phase: getting the client’s approval. Tunnels are essential for the development of megacities like Hong Kong where space is in short supply and traffic jams are everywhere. The authorities have developed highly competent state advisory groups and hired talented civil engineers for such projects. They’re all experts in their fields, but they know little about diving. When Armin proposed to dive to the TBM, they said: “We understand, and we’ll use the Black Pool air tables that the British left behind.”
The Black Pool tables that were used primarily in between the two wars bent a lot of divers. Professor Walder built an entire scientific career by publishing on osteonecrosis after the tables. We said we could do better. The immediate reaction of the clients was: “What! How would you dare to compute new tables? Are you going to bend workers?” Armin entrusted me to explain.
Science, which is based on knowledge and data, aims to elaborate on theories that could provide explanations and make predictions. Decompression theories hold until they are invalidated by the emergence of new data or concepts that better explain the observed phenomena. Dissolved gas models have been pushed into the dustbin by bubble growth models; the latter became obsolete after publications on microparticles, and oxy-inflammation theories, and so on.
Designing decompression tables is technical, high stakes activity. It relies on science, but its goal is to create a practical solution to an operational problem. It epitomises Socrates’ philosophical dilemma of action: how to achieve just and moral goal, with limited experience and knowledge.
Diving engineers do that every day. Not because they are immoral, but rather because they have a solid method. After considering the many layers of complexity, they grant themselves the right to simplify the reality: After all, what could the impact of gene activation or a biomarker expression on the duration of a given decompression stop? Divers may come in all sizes, shapes and ages, but there is only one rate of ascent for the chamber. Then, the engineers proceed by validating their assumptions one by one. They monitor divers to test the tables. If a problem occurs, they process rapidly and react appropriately. If things go smoothly, their assumptions are validated. Diving engineers are imperfect (they use lousy approximations) but they have a perfect method (they believe that small changes will produce small effects that they can evaluate by monitoring).
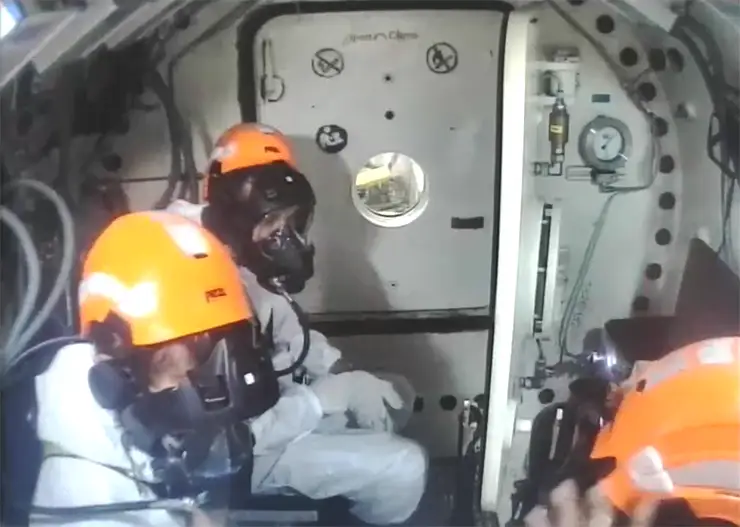
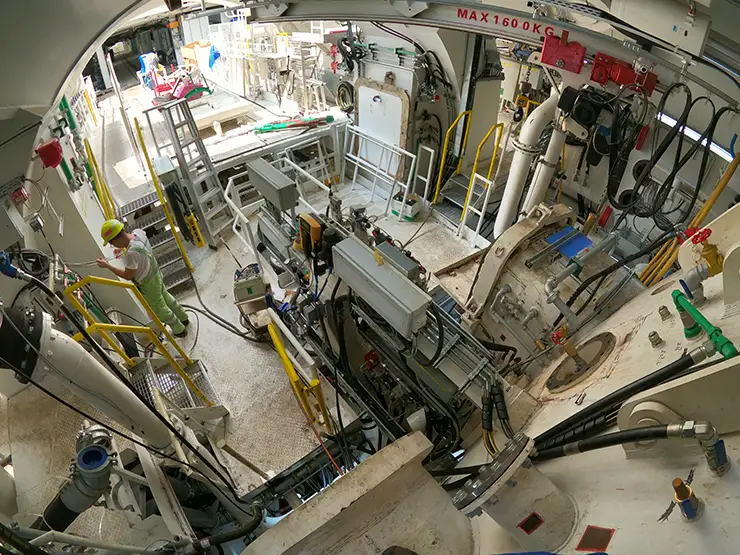
We won our case. The clients agreed to use the new trimix tables provided they would be scientifically monitored and their safety performance documented.
The next question was: How about these specific tables? Table designers have few tools available in their box: decompression time, decompression profile, and gas switches. Decompression time is obvious, but profile is a more difficult concept to catch. Just remember Haldane.
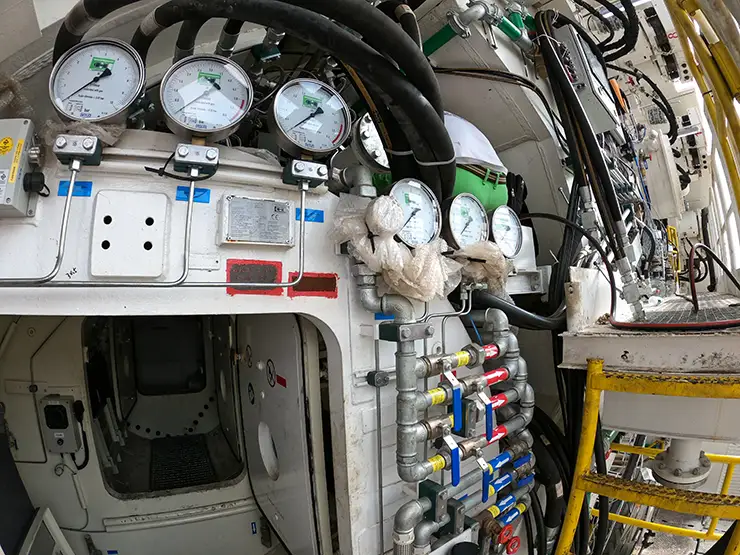
Developing Deep Diving Procedures
At the turn of the 19th century, the Royal Navy sought to develop “deep” diving procedures for operations deeper than 30 m/100 ft. The Admiralty first contracted Hill, who was a specialist of his time and believed in “uniform decompression,” a linear ascent used by sponge divers and tunnel workers.
Hill decompressed himself from 60 m/200 ft to prove this first concept: decompression time is critical. Provided the ascent time was long enough, they thought that divers could decompress from any exposure. But linear decompression proved to be disappointing and, in 1906, after a series of decompression sickness (DCS) cases, the Royal Navy contracted John Scott Haldane, the world’s reigning expert on respiration.
Haldane kept very busy during his time in the Navy. He had first resolved the problem of CO2 retention inside the helmets and set rules for the number of sailors required to operate the pump, depending on the depth, to ensure a sufficient air flow. He had then built a chamber to tackle the decompression issue, using goats as an animal model and articular pain as a clinical endpoint. After 42 goat dives, he finally published his famous paper “The Prevention of Compressed Air Illness” in 1908, which presented his model and two sets of tables (one for “ordinary limits” and one for extended exposures), with entry depths down to 34 fathoms (62.1 m/204 ft). We even suspect that he dived his own tables because he added a paragraph on a method of lacing the drysuit legs.
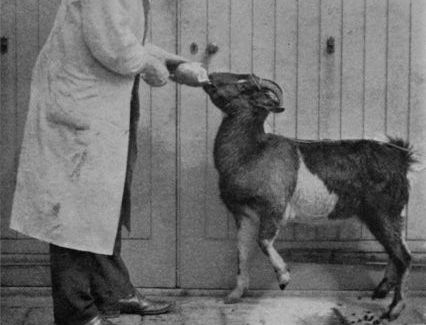
Haldane perfectly understood his colleagues and their scientific work, but he enjoyed challenging them by introducing an exponential gas exchange model and a simple safe ascent condition based on supersaturation. This proved very successful because the exponential response of tissue compartments requires an initial pull to create a gas gradient.
Hence, decompression starts with a rapid ascent to a first stop and follows with slower ascent by “staged decompression.” The concept of the ascent profile emerged. It suggested that, for the same decompression time, different strategies could be used to conduct a safe ascent.
A good analogy is planning a half-marathon that you reasonably expect to finish in two hours. You could suffer through a fast pace on the first 5 km to create a gap and progressively slow down for the rest of the distance. Alternatively, you could save your resources by starting slowly and running faster at the end of the race. You could decide on which strategy to choose based on the conditions: if you knew that the last 3 km included a steep incline, you might choose the energy preservation approach.
Similarly, the first stop depth sets the pace for the rest of the decompression; a shallower first stop with a high ascent amplitude would require a longer 3 m/10 ft stop; a deeper first stop with a moderate ascent amplitude would allow a shorter 3 m stop. The first stop depth roughly controls the ascent profile.
Once divers chose their decompression procedure, they could optimise other elements of the dive. For instance, the decompression strategy might vary from air surface decompression tables to in-water decompression, from open circuit dive to closed circuit rebreather dive with a constant oxygen setting. This is why tables used in military diving for mine warfare had different profiles than the ones used in commercial diving for bolting two pipe flanges.
The next question was how to assemble a decompression profile. I had learned the recipe when I worked at Comex.
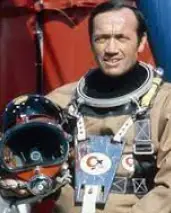
At the turn of the 80s, HG Delauze, the boss, knocked on the door of Comex Hyperbaric Centre and said, “I had a dream. I saw the development of deep diving in Brazil and in Norway. Could you please select 100 Comex divers to prepare for upcoming projects like these.” He was right, but the scientific team was reluctant. They answered that yes, they knew how to build a scientific package for testing divers against HPNS, but the test lasted for 90 minutes and the minimum depth to consider for HPNS was 180 m/590 ft. The boss just suggested we design a 180 msw/120 minute table and then parted.
This had never been an easy dive, and the first attempts turned into disasters: No decompression algorithm available at the time would work. Pressed to do something, the person in charge of calculating the decompression eventually plotted decompression schedules on millimetric paper sheets. Not a good idea; we all have done it before, and it just adds confusion to the overlapping profiles.
In a desperate attempt, he tried a semi-log paper sheet and then a miracle occurred: On a semi-log plot, decompression profiles look almost like straight lines. Hence, using a ruler, a pencil, and a semi-log sheet, he designed a decompression for a 120 minute dive at 180 msw/ 590 fsw dive that proved extremely successful: 48 divers in a row went through the table without any DCS symptoms.
Table It
Figure 1., shown below, displays the famous decompression schedule. The X-axis represents the stop depth in msw and the Y-log axis directly displays the stop time in minutes. Note that assuming constant 3 msw stop increments, the stop time represents a decompression rate (the time it takes to travel 3 msw). As everything is exponential in an Haldanian model, no wonder that the profile appears linear in a semi-log sheet.
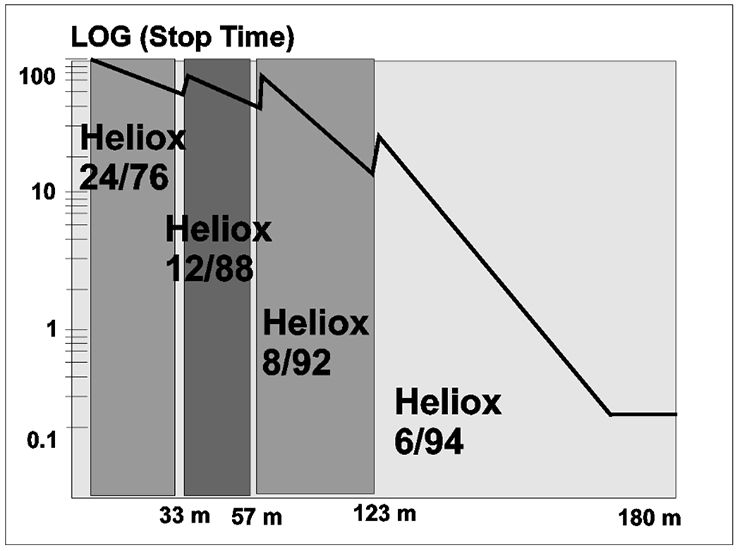
I have found this semi-log plot very convenient to handle profiles. It expands the initial part of the decompression and permits immediate evaluation of the rate of ascent and the first stop depth. It also allows one to compare decompressions in the same diagram regardless of their duration: for instance, different bottom times for the same bottom depth. After some practice, one learns that:
- The slope of the line is related to the percentage of oxygen. The higher the oxygen content in the mix, the faster the ascent.
- A change in decompression mix accelerates the ascent rate. A decompression with several mix changes displays a sort of saw-tooth shape.
- The longer the bottom time, the slower the ascent. Two dives at the same depth, but with different bottom time, display parallel lines.
Try the semi-log plot on any of your deep profiles and you will see the linear segments appear for each mix. This is the best way to represent and compare different decompression profiles.
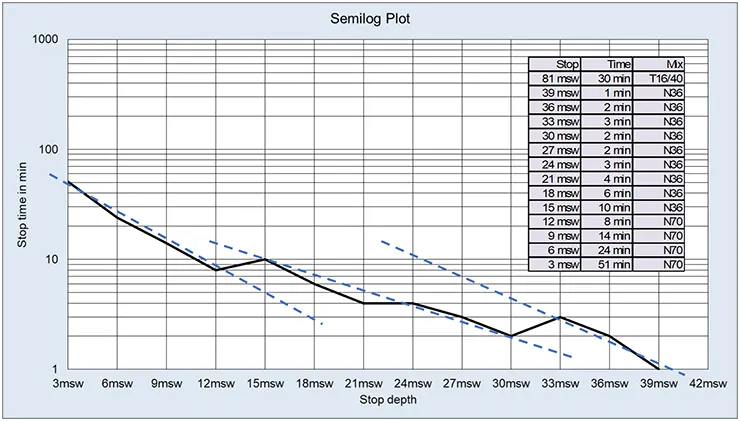
Black Box Modeling
Now, consider the Gradient Factors.
Scientifically, Gradient Factors represent a very low level of actual knowledge, but they do incorporate massive accumulated experience. For instance, I met Xavier Meniscus recently, and he gave me all his Gradient Factors he used for his deep dives. It represents a large and unique experience and I could, in theory, be diving to 200 m next weekend at Font Estremera. In practice, his combinations of GFs summarise his experience of Haldane’s concepts of decompression profiles and duration:
- The GF low sets the first stop depth. It defines the profile.
- The GF high sets the last stop duration. It more or less defines the decompression duration.
Gradient Factors refer to a black box model which corresponds to ground zero in terms of mathematical modelling. But this black box only requires two parameters to produce an infinite variety of decompression times and profiles; some of them might even be good for you.
This brings me to the final statement, which is hard to admit for a person who spent his life designing decompression tables: there is nothing like the ultimate decompression algorithm but rather various strategies based on different experiences. Thus, mathematical modelling is not any better than using Gradient Factors. Artists and diving engineers tend to fall in love with their models. I, therefore, used my algorithm to compute the tables, but I am ready to admit that one could obtain the same result using GF 65/70.
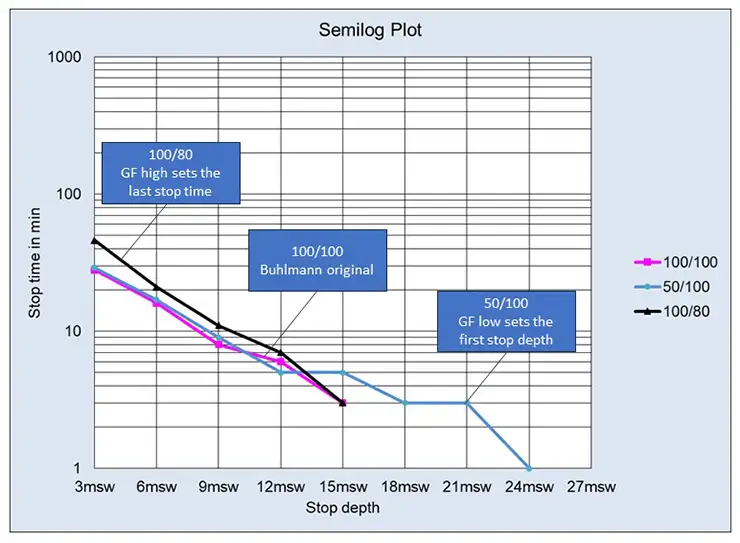
Trimix, The Cinderella of Diving
Decompression algorithms keep the diver spending time at stop depths to control their slowly varying gas gradients. The only thing one can do to increase these gas gradients and accelerate decompression is to introduce oxygen or inert gas sequencing. And this is the beauty of trimix decompressions.
For the TBM dive, the recipe first included a massive dose of oxygen breathing. This is possible because the TBM has a chamber fitted with a BIBS (built-in breathing system) that permits oxygen breathing via a mask at 12 m/40 ft. It’s just a matter of introducing interruptions in the mask sessions (25 minutes on, 5 minutes off) and checking the ESOT oxygen toxicity index. After all, Dr. Lambertsen (the master of oxygen toxicity and the father of the UPTDs) used to say, “Oxygen is good for you! Better to cope with the effects of oxygen toxicity than decompression sickness.”
To this I added a personal touch introducing an intermediate decompression mix before oxygen. This is the trick in trimix decompressions. It first raises the PO2 during the ascent, and increases the gas gradients. It also provide the possibility to change the inert gas nature. Options are nitrox or heliox. Before screaming about isobaric counter diffusion, just refer to treatment table Cx 30, which very efficiently treats a bent air diver with 50/50 heliox. Forget the details and concentrate on the idea: helium treats nitrogen bubbles; heliox is the right choice after a trimix dive for an intermediate deco mix.
The decompression becomes a double push/pull game. Breathing heliox as a first deco mix pushes the nitrogen out and pulls helium in, which is fine, as helium is an easy gas to decompress. It also helps in terms of isobaric counter diffusion. Then after, at 12 m/40 ft, oxygen pushes helium out and finishes the decompression job.
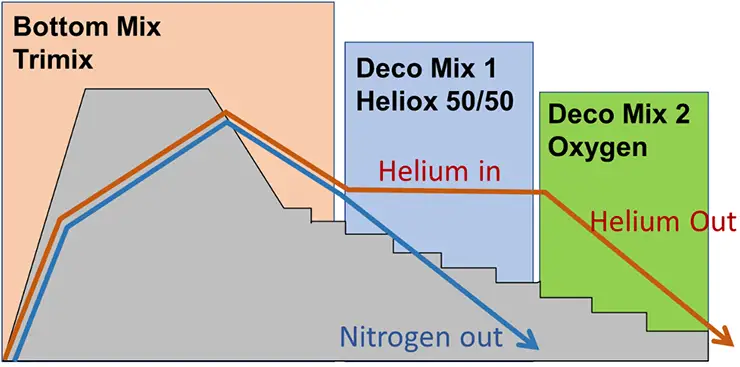
So, our duo worked as the two faces of Janus. I provided the tables, and Armin decorated his dive control with sophisticated trimix panels.
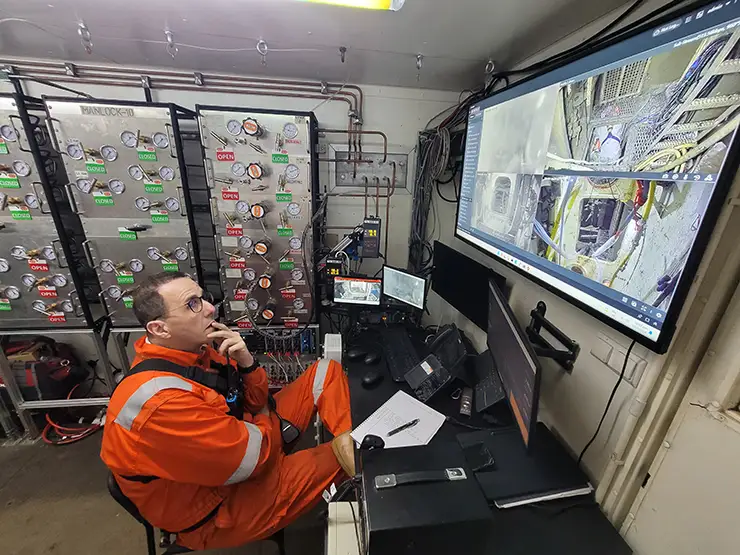
This being done, the last phase was building a scientific team for diver monitoring. We started the project naively saying, “You can only improve what you can measure,” and then realised that the difficulty was defining the measures of a good decompression. Alf Brubakk said decompression is a stress; in fact, a multiple factorial stress. You start with immersion, heat and cold, dense gas breathing, hard work, dehydration. You continue with the physical implications of all the possible bubble scenarios. You finish with microparticles, oxidative stress, neutrophil activation, and endothelium damage. You then realise you need friends in the scientific world to help you assess the decompression stress.
Long story short, we used PhysioPad, which is a simple software on a tablet running psychomotor tests and questionnaires. We teamed up with the University of North Carolina—who provided deep learning technology to automatically analyse bubble signals—and the University of Padova—who had expertise tracking inflammatory biomarkers in saliva and urine. We added a bio-impedance, which we believed could indicate building of the decompression fatigue by tracking cell membrane function and the water movements. We summarised the results in a neat radial diagram.
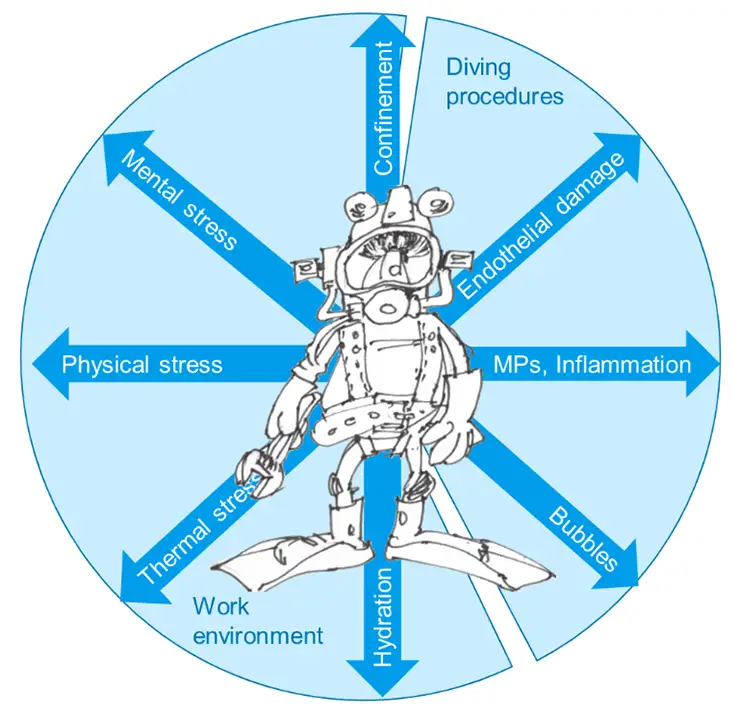
During the next two months, anytime I got Armin on WhatsApp, I could hear the BIBS supply regulators singing in the background. Finally, Armin succeeded in closing a damaged hatch at 9 o’clock and got the TBM dry. The rest was easy, and they managed to cut a few more meters of tunnel and park the TBM in granite where they could repair it. It was then, and only then, that Armin told me his story.
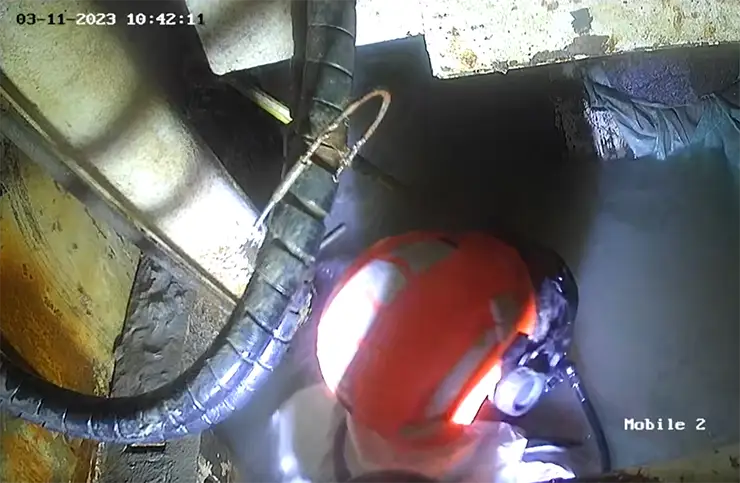
The first dives were difficult. The front chamber of the TBM was a mess of steel beams and hydraulic hoses in narrow passages just the size of the Superlite 17 helmet. The cutting wheel was 14 m/46 ft in diameter, and the visibility was liquid mud. But Armin knew all the bits and pieces of it and could not resist the dive. So, he dressed, immersed, and progressed into the dark maze carefully dragging his umbilical behind him. And suddenly, there was a gust of gas (a leak) that sent him flying and left him upside down somewhere in the structure. He cooled down to assess the situation. They tried to pull the umbilical in, but it was stuck in a narrow groove that would not permit any movement. He then started methodically exploring his environment. The surface team added a little thrill by telling him, “You got 15 minutes left on your table.” Suddenly, he touched a bolt that he immediately identified. Knowing where he was, he managed to return, despite his stuck umbilical.
Trimix is the Cinderella of diving; while it used to be the “poor man mix,” it has now become the most advanced diving mix and the ultimate weapon for difficult decompressions.
At the end of these stories, these are the lessons learned: It takes time to become a tunnel intervention manager, and Armin was happy he had been a cave diver in one of his past lives. Trimix is the Cinderella of diving; while it used to be the “poor man mix,” it has now become the most advanced diving mix and the ultimate weapon for difficult decompressions.
DIVE DEEPER
BOUYGUES CONSTRUCTION (VIDEO)-HydroKarst Group: Saturation works for maintenance operations in TBM – Hong Kong. Bouygues-Hydrokarst trained 50 hyperbarist technicians to live and work in saturation for maintenance operations in the TBM cutter head.
YouTube: Hong Kong Built A $6 Billion Underwater Tunnel
InDEPTH: Decompression, Deep Stops and the Pursuit of Precision in a Complex World by Jarrod Jablonski
InDEPTH: Caisson and the Brooklyn Bridge by Jarrod Jablonski
DTIC: Analysis of DecompressionTables Calculated by Non-U.S. Navy Means (Black Pool Tables) by Peter O. Edel
aquaCORPS: DIVA, The Lady of Commercial Diving by JP Imbert (1993)
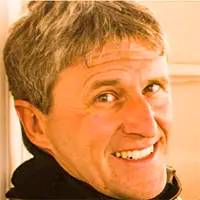
JP Imbert spent 19 years at Comex, a leading diving company, as Diving Manager. He was involved in the Comex research hydrogen dives and computed the French MT92 tables. He participated in the Norwegian deep projects of the 80s and developed the Comex procedures for saturation to 300 m/1,000 ft in Brazil. He then turned to technical diving. He became an IANTD instructor in France and trained trimix and rebreather divers for 10 years. JP is now back to the offshore industry as a diving consultant, editing diving manuals and decompression procedures for large North Sea companies. He has steadily kept publishing on decompression modelling and recently on diver monitoring.
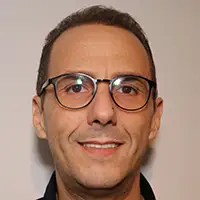
Armin Sidali is the hyperbaric manager for Bouygues Constructions, a French company. He became a commercial air diver in 1997 and, in 2003, a mixed gas diver, closed bell (saturation) diver. He worked as a commercial diver for the oil and gas industry. He started his tunnelling career in 2009 in Barcelona, Spain, where he used the first of JP Imbert’s trimix tables using heliox 50 and oxygen in decompression. From 2015 to 2018, he headed saturation operations in tunnelling in Hong Kong for two, 14-m diameter, subsea tunnels totalling 18 saturation cycles (4 weeks each).