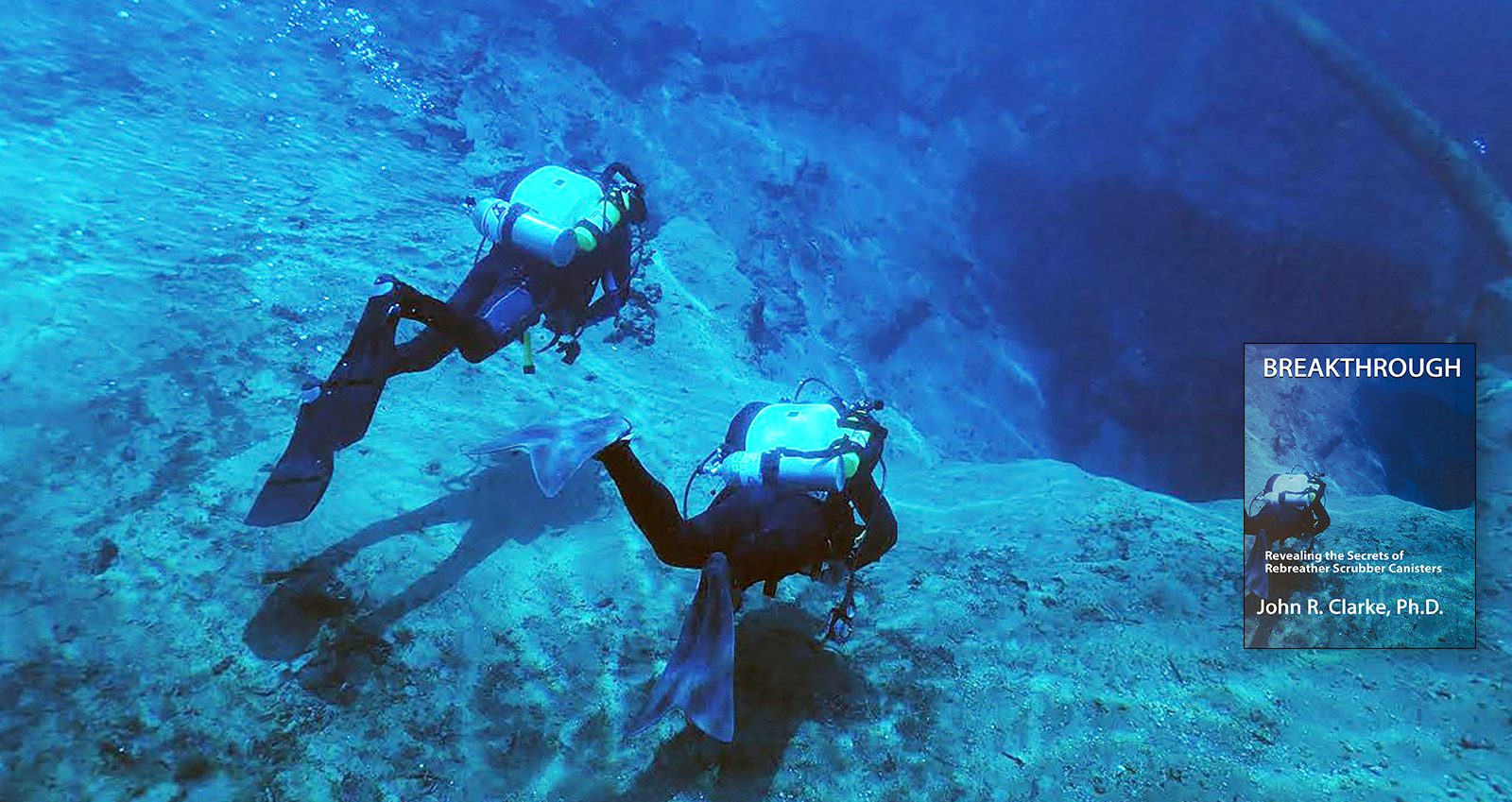
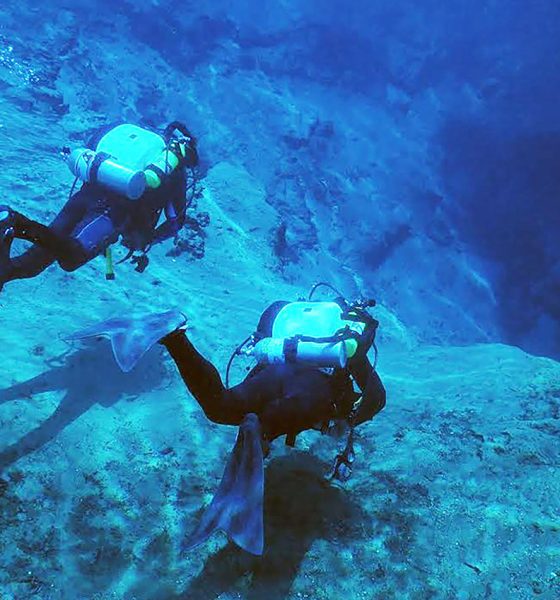
Equipment
The Secrets of Scrubbers
Dr. Clarke’s geeky new monograph on the inner workings of rebreather scrubbers represents the culmination and synthesis of more than three decades of Naval research, as well as the personal research of the retired scientific director of the Navy Experimental Diving Unit (NEDU) into stochastic systems. As such, it is arguably a MUST READ for serious rebreather users. Here marine scientist and rebreather instructor Jeffrey Bozanic dives into some of the important details of Clarke’s book and the operational questions it seeks to answer. Feed your head!
By Jeffrey Bozanic PhD. Header image courtesy of Bernie Campoli.
Let me begin this book review of Breakthrough: Revealing the Secrets of Rebreather Scrubber Canisters by John R. Clarke with my conclusion. This is one of the most important works available to rebreather divers. It dispels many unsafe practices and provides a base for making informed decisions. This monograph is a “must read” for all serious users of rebreathers, all rebreather designers, and all rebreather manufacturers.
I began diving rebreathers in 1988, and since that time have accumulated in excess of 2,500 hours in a wide variety of open water conditions. Early on in this time, I began wondering about the truth of “best practices” that were taught to me (and that I have since been teaching), as access to quantifiable and repeatable data was lacking in our civilian/scientific diving communities.
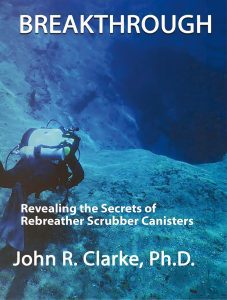
I often wondered about scrubber canisters, one of the intrinsic critical components in any rebreather. How well do they work? What can make them fail? How long will they really last in the environmental conditions I use them in, as opposed to how they are tested? What is the best method for packing canisters? Can canisters be safely used on a dive, stored, and reused? If so, how long may they be stored? Should we modify use time based on such storage? What are the impacts if the canister becomes partially flooded? Are there practices which will extend absorbent efficacy, either during or between dives? Can I modify canister times based on my expected work rate, compared to the work rates simulated during testing? Does my personal size (240 pounds/110 kg) and fitness level suggest that I modify canister use time compared to test standards? What are the implications of using one absorbent grain size versus another? How much does ambient water temperature impact absorbent efficacy? How can I modify my personal use time based on that? And this is but a partial list of questions I have had.
Sure, we all have stock responses to many of these concerns. “Well, we know that absorbent will last longer in the 84oF/29oC water in which I dive, so I just double the manufacturer’s suggested time.” Or “If you bag and label your scrubber after use, you may use it on a future dive.” Most of these responses are based on anecdotal experience or data, so they may not be completely without merit. And most seem to make sense if we think about them hypothetically. But none have been adequately tested or modeled from a scientific perspective.
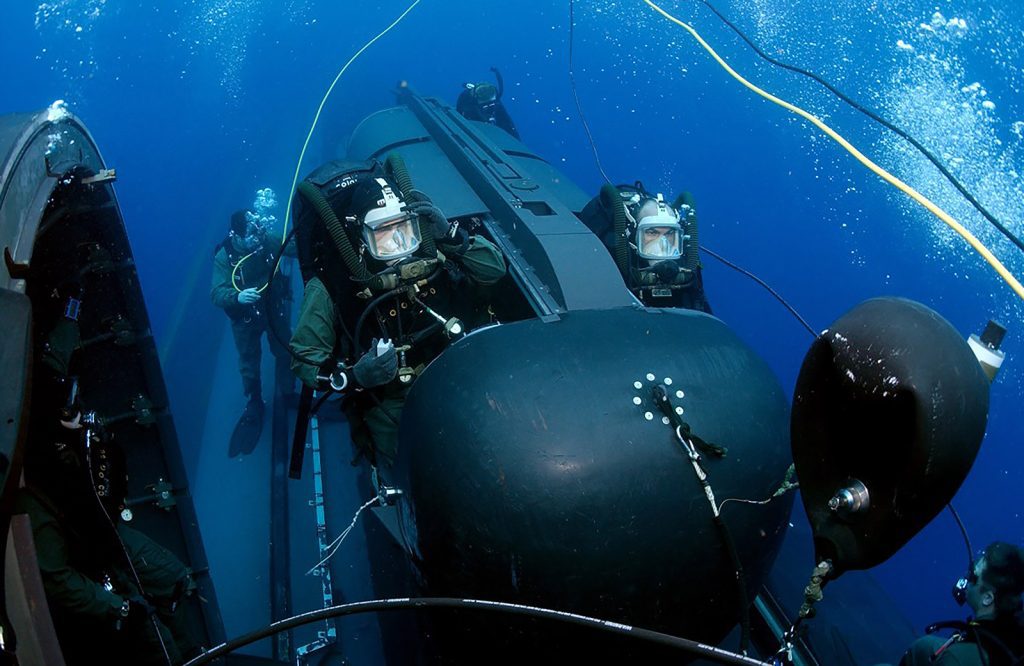
It’s The Data, Stupid
I mentioned earlier that, “access to quantifiable and repeatable data was lacking in our civilian/scientific diving communities.” In much of the research that I have done, there were indications that such data may have existed in the military community, specifically at the US Navy Experimental Diving Unit (NEDU), where such questions are typically researched to support military divers in completing their missions successfully. However, as a civilian, I did not have or had only limited access to such research documentation. I found this to be extremely frustrating. And it left me curious as well: What do they know that I do not?
Enter John Clarke. John was the Scientific Director at NEDU for 28 years. A physiologist by training, he found himself working with divers, engineers, and mission planners on projects or research designed to validate options for classified operations. Much work revolved around rebreathers, including scrubber canisters. In fact, before this book was published, the manuscript had to be reviewed and approved by the Department of Defense, an uncommon process for most publications, to ensure that it contained no classified information. While there were minor edits made, they allowed the publication to go forward.
You see, John had developed most of the model upon which much of this book is based during his personal time, after hours and at home outside the workplace. While he used the fruits of his labor at NEDU, it was not a NEDU project. But the reality is, John’s work benefitted from his NEDU projects and experience, and the research at NEDU benefitted from John’s extracurricular labor.
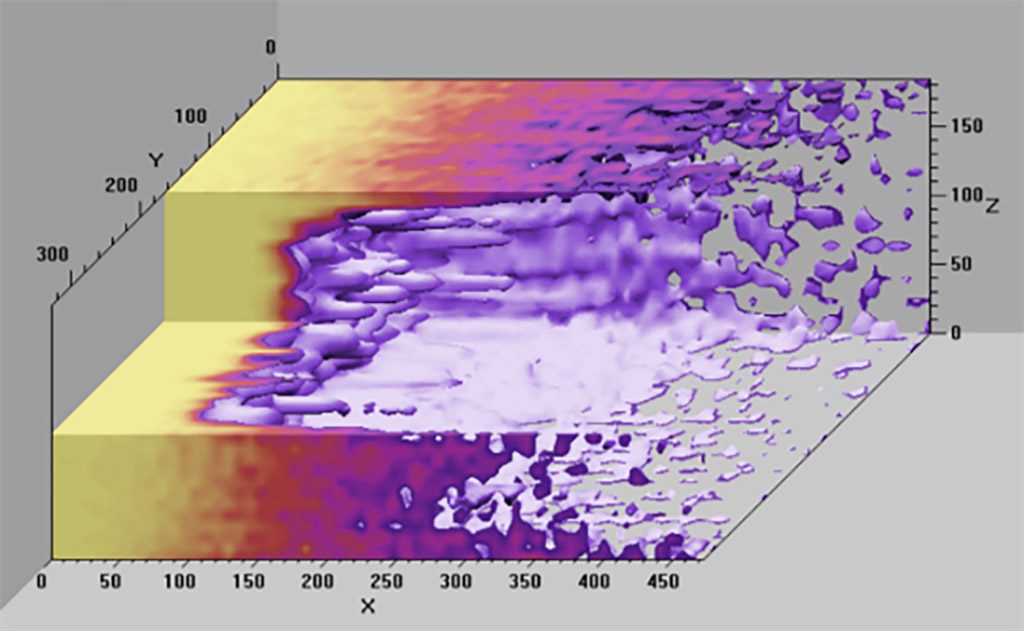
To understand the benefits (and limitations) of Breakthrough, you must first understand the differences between some basic concepts: hypotheses, anecdotal information, experiments, and modeling.
Hypotheses, Anecdotes, Experiments, And Modeling
A hypothesis is an idea or thought that you believe could be true. There are two basic types of hypotheses, those that you believe may be correct (true), and those that you set up as “straw men,” hoping that by proving them incorrect that the obverse must then be true. Most people inherently understand the first type of hypothesis. In fact, some people look at some of these hypotheses, and because they seem so inherently reasonable, often consider them to be “true.” An example of this might be the statement that, “The more absorbent you use in a rebreather, the longer you can remain underwater.” This seems reasonable, right? Yet, what about the case in which the ability to dive longer is limited by oxygen supply, and not by absorbent? Or when, even though you have more absorbent, the resultant increase in breathing effort caused by the increase in gas travel path through the absorbent bed leads to increased breathing effort, increased carbon dioxide generation, and consequently reduced dive time? We should not confuse a hypothesis with “truth.” Unfortunately, this is relatively common in the civilian diving world. It requires validation to prove a hypothesis.
Many people believe they can validate a hypothesis with anecdotal data. This is information which may have been observed but may not have been rigorously structured. A common argument heard in the recreational diving sector is, “Well, I did it, and I’m fine.” That argument is often used to justify further deviations from recommended guidelines. This normalization of deviance may continue until a significant adverse incident (such as a fatality) occurs. Even short of such an event, using anecdotal evidence is skewed reasoning.
Consider the example of a rebreather diver using a specific rebreather, the manufacturer’s use guidelines state that their rebreather may be used for three hours in 40oF/4oC water at a VCO2 (metabolic carbon dioxide production rate) of 1.6 LPM (liters per minute). The diver recognizes that their typical workload during a dive produces much less carbon dioxide than that, and that they dive in the Caribbean Sea at much warmer temperatures. So they hypothesize that they can dive longer than three hours, and “test” that hypothesis by conducting a dive of four hours. Surviving that dive, their next dive is five hours, and so on until they are diving their rebreather for ten hours on the scrubber. Obviously, the manufacturer must have been overly conservative in their guidelines, correct?
The fallacies with using this type of anecdotal evidence to “prove” their hypothesis are many. The diver’s workloads are not consistent during the dives, and results from one dive will not match another. Water temperature varies seasonally, with depth, and often with location, due to currents and other factors. Depths were almost certainly not constant, impacting gas density and scrubber efficacy. Finally, there was no means of objectively determining the partial pressure or percentage of carbon dioxide in the breathing loop at any given time, other than, “I felt OK.”
My personal research has shown that divers will not notice a 5% CO2 level in a breathing loop over a short time, where 0.5% is considered a “safe” limit. All we learn from the body of anecdotal evidence from our diver is that he survived what may have been hundreds of hours of “testing” and “proof” of his personal guideline.
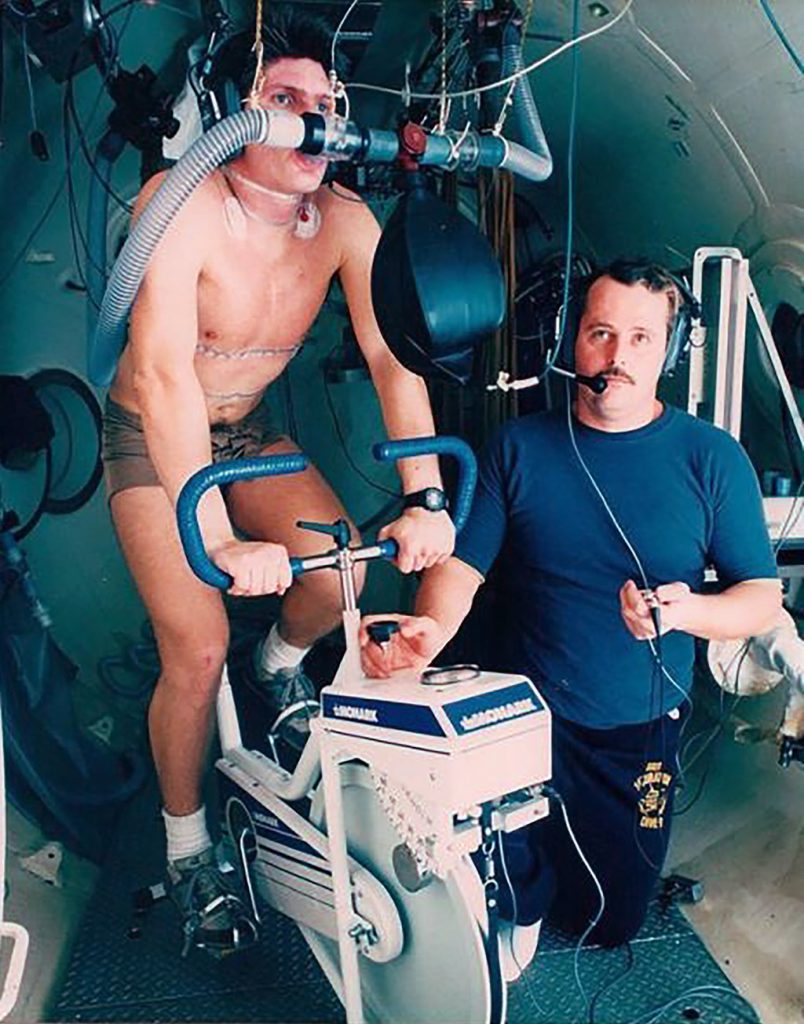
The only way to prove a hypothesis is through experimentation. Experiments are tests in which all variables are tightly constrained, so that the impact of any single variable (like the time a scrubber will last) is well understood and defined. If conducted properly, the results are consistent and repeatable. In the previous example, multiple trials of duration would be conducted, constraining water temperature to 84oF/29oC, depth to 130 fsw/40 msw, VCO2 to 1.2 LPM, and having a way to actually measure when PCO2 reaches 0.5% SEV, as one example. We would then need to run this same set of constraints multiple times to see that we are getting consistent results, before changing one of the independent variables and repeating the experiment again.
The problem with experimentation is that it is time consuming, and expensive. Also, some observations we might wish to make (like seeing the inside of a scrubber real time) is not possible. Modeling allows us to circumvent these issues by establishing a set of mathematical equations that simulate what occurs in real life. This would allow us to predict results if we dove in those actual conditions. Modeling is limited by how well we can establish the mathematical formulas that we use for predictions, the granularity (fineness) of the model, and the processing power and time available. Models are validated and revised based on experimental results and occasionally anecdotal data.
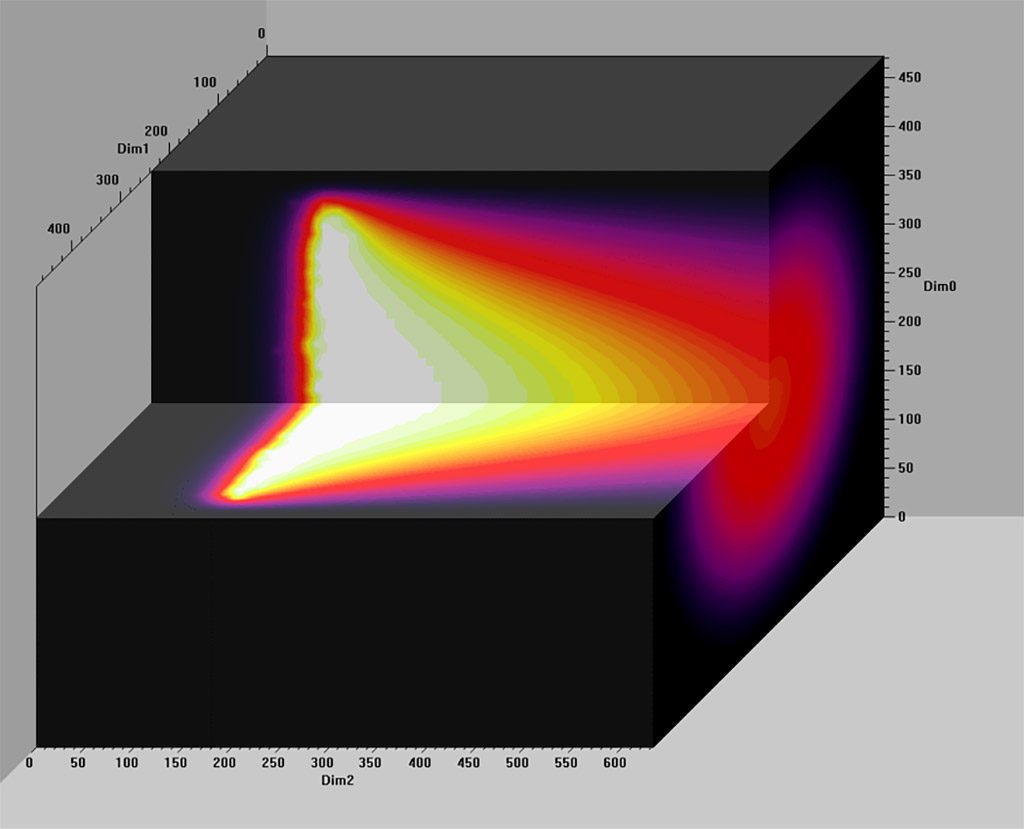
Breakthrough uses all of the above approaches for considering scrubbers. However, much of the predictive information and discussion arise from models that John has built, which have consequently been refined and improved upon using the experimental data from his years of research at NEDU. While this information is new and interesting, it did not answer all of the questions I asked above, and may leave you wondering with your own specific questions. That said, I still found the book illuminating, and in some cases downright scary. The remainder of this review I am going to spend highlighting a few concepts and results from the text that I found particularly engaging.
Before I begin, one last observation: John’s goal was to provide relevant information, not just for divers, but also for theorists, engineers, and physicists. The book is based in large part on math. I have had several years of calculus and statistics in the course of my academic studies and still found some of the math to be above my head. Do not let that dissuade you from reading it. Even if you do not understand the math, John’s discussion generally highlights the importance of what he is trying to explain with the math, and the diagrams help as well. If you get to a part of the manuscript where the math is daunting, just skip over it and resume your reading when you get past the numbers.
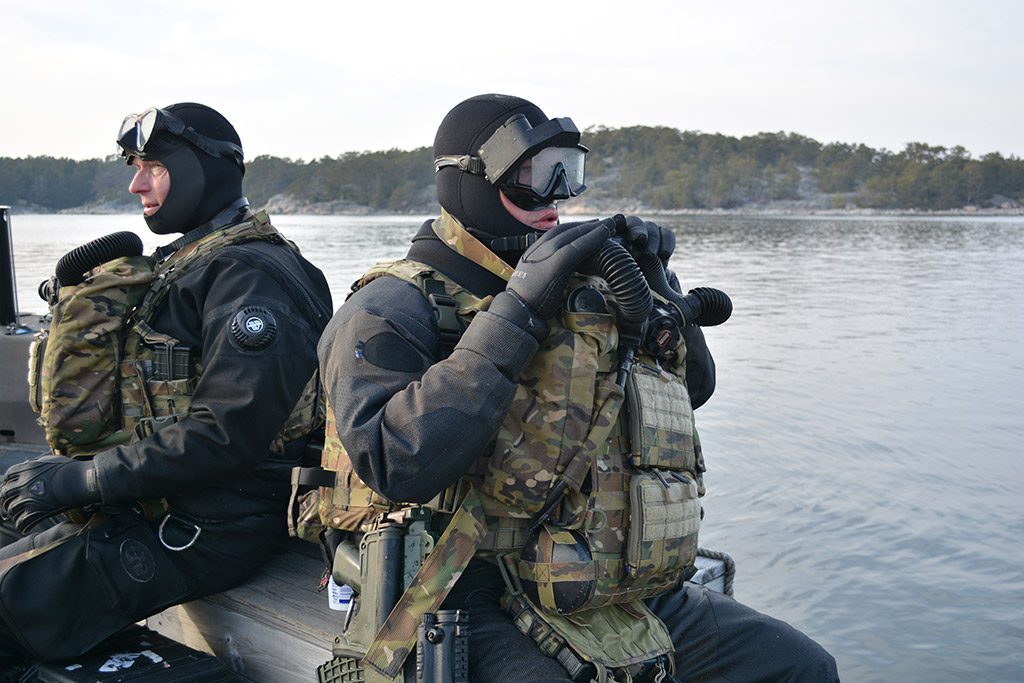
A Study In Variability
In many respects, the book is a study in variability. How does the real world vary from theory? How do variations in constraints impact scrubber performance? What role does environmental variability play in scrubber efficacy? When may small, seemingly inconsequential variances have a large impact on final results? How does physiological variability impact scrubber performance? And what should we do to increase dive safety based on this information?
Revelations began on the very first page of the text. Examples here, and on pages 3 and other locations, discussed problems with the actual absorbent products provided by different manufacturers. Contaminated absorbent, mislabeling, grain size variations, and extrusions could or were all impacting scrubber performance for divers. I had never considered that there could be defects in the actual absorbent that were undetectable by cursory visual examination.
An engineering concept that John stressed in the book and that I had seen before—but was not really familiar with—was propagation of error. While mentions of it are made throughout the book, the most concise discussion was on pages 45-52. In this example, the potential impact of physiological uncertainty and conversion of metabolic oxygen consumption to carbon dioxide by any given diver, means that breakthrough could occur much sooner than the published limits, in this case, it’s likely to be a 13% shorter duration. What John is saying is that during manned dives, breakthrough can occur well before unmanned test limits indicate.
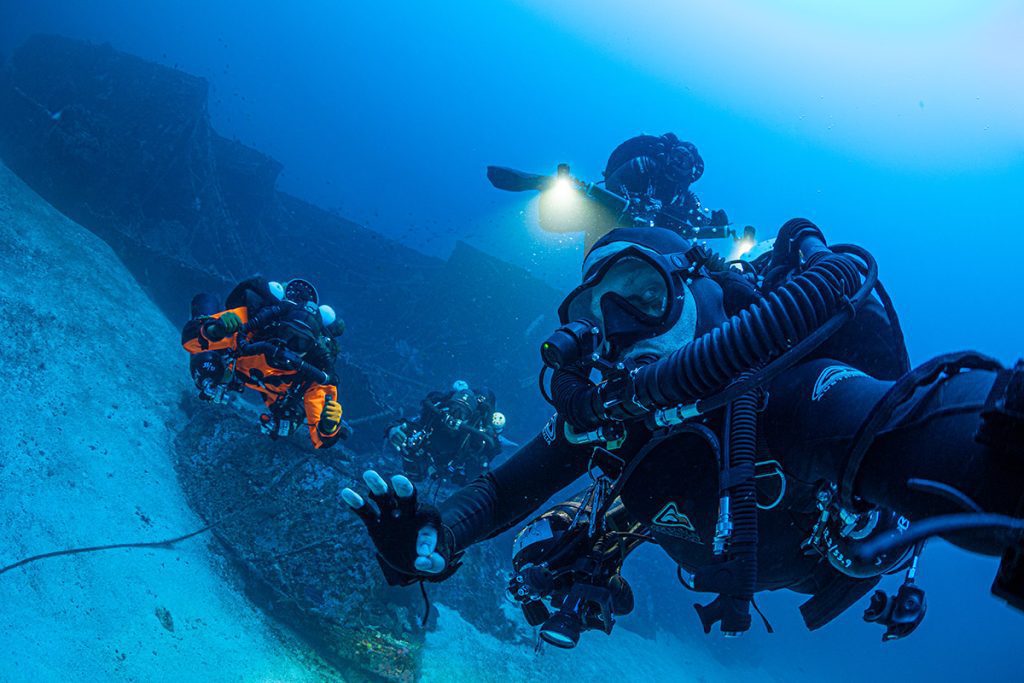
This is scary! Putting it another way, in 17 out of 100 dives, the PCO2 would exceed 2% SEV, (a generally accepted “safe” value when diving a helmet, but not α rebreather) within the published time, a value four times than expected. In actual field practice, we make the exact opposite assumption, based on differences between test water temperature of 40oF/4oC and our dive environment temperature (warmer), and anticipated VO2 and VE being less than test standards. The point here is that I have always regarded published canister limits as conservative and to be regarded as a starting point for determining a reasonable dive time on a scrubber. This may not be true. Later in the same section, using a different set of covariance data, he demonstrates that a diver may reach a 2% SEV level as often as one dive in five (about 20% of the time) due to propagation of error effects.
Having worked with statistics to evaluate my own research data on many past projects, I am quite familiar with confidence intervals and prediction limits. However, I had never considered them with respect to scrubber performance. The same figure indicated a reduction in time of 20% at some temperatures, when a 95% prediction limit was considered. Page 50, Table 4, provides data on high and low COV and indicates that there is a 1 in 42 chance of breathing 6.3% CO2, when aiming to conclude a dive at 0.5% CO2. I found this astounding. I think John summarized it best on Page 54, “Being able to safely dive to a single published canister duration, such as 180 minutes, is a fantasy.” This discussion also indicates to me that we in the diving community would be much better served if the manufacturers providing us with canister limits also provided confidence limits with those figures.
Respiratory exchange ratio (RER) is a measure of how much CO2 is produced based on the amount of oxygen consumed. I had always considered a value of 0.8 to be typical for this, although I was aware that it varied, particularly with diet. However, the Navy uses a value of 0.9 for their standard, and some experiments conducted by the Navy resulted in a RER of 1.07 (Page 16, “VCO2 was 1.4-1.9 LPM, and VO2 was 1.21-1.77 LPM.”) In other words, the divers were producing more CO2 than they were consuming oxygen! This has obvious implications for the rebreather user.
The Power of Modeling
In Chapter 5, John begins the discussion of the model he has developed for predicting scrubber performance. He explains the parameters and how the model evolved as he used it. The diagrams are quite interesting and are an important stepping stone to understanding the discussion in Chapter 6 on cold soaked scrubbers and other applications.
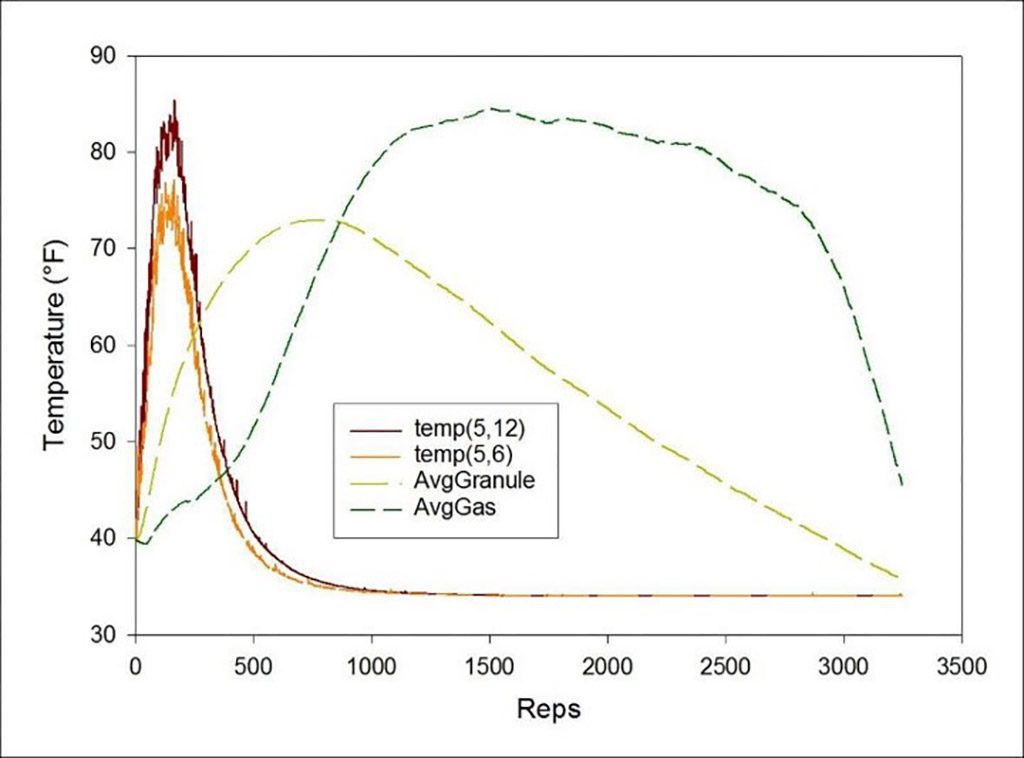
One section titled “Decoupling Work and Ventilation” on Page 75 considers hypoventilation. This is a common practice as many experienced open circuit divers often train themselves to hypoventilate (“skip breath”) as a technique for conserving gas. This practice may be contraindicated when using rebreathers, but the habit is so ingrained due to muscle memory that the diver is unaware of it. In the experimental setting discussed on the following page, the practice directly resulted in the diver passing out while under water from high end tidal CO2. This may, in fact, be related to “deep water blackout” that has led to fatalities in some deep water cave dives.
One of the things I needed to keep in mind was that these chapters dealt with modeling, not actual dive operations. I found it enticing to think of applying this information to my personal diving habits. For example, on Page 95, he states, “The simulation ran about 3.5 times longer in the warmer temperature before canister breakthrough.” It would be easy to use this as justification of extending canister use to 3.5 times the manufacturer recommended limit. However, John then goes on to caution the reader that these are model results, and should not be used to plan real world dives. That warning should be kept in mind while evaluating all of the modeling results. Modeling indicates possibilities that then need experimental validation prior to adopting for actual dive applications.
Page 97 begins a discussion of Macroscale Variability. Translated, John is referring to whether the canister is completely filled with absorbent. Practically, what is being discussed is how well the canister is packed with granular (loose) absorbent, a critical skill in any rebreather training class. Unfortunately, we have no means of objectively determining if we have accomplished this vital task adequately. Perhaps weighing the final packed canister is the best alternative, but few divers do this on a regular basis. However, John shows in Figure 70 that with only a 99% fill compared to a full fill, there is a possibility of as much as a 35% loss in duration before breakthrough.
This is an astounding number, and emphasizes how important proper canister filling is to the real world diver. It is also a strong argument, in my opinion, for the utilization of prepacked canisters (like those used with Poseidon rebreathers), or solid absorbents (like Micropore’s ExtendAir cartridges).
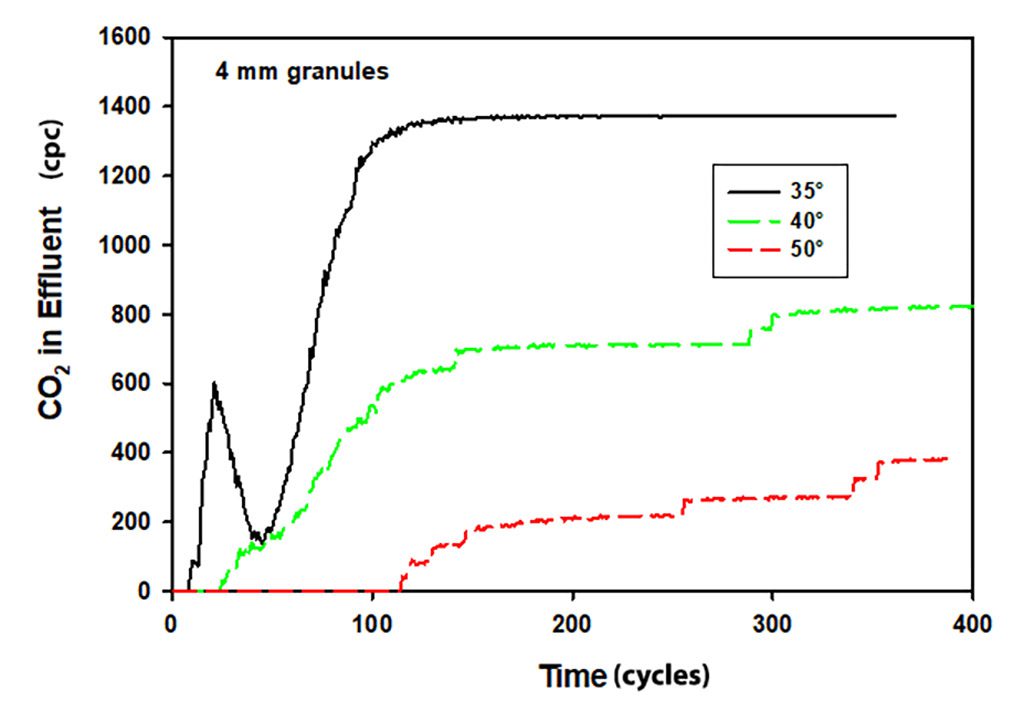
I have always believed that long pre-breathe times (>30 seconds) were unnecessary as part of pre-dive preparations, given water temperatures of 50oF/10oC or warmer. The data plotted on Page 120, Figure 88, seems to support that hypothesis. What I did not expect, however, was his discussion on Pages 119-122 highlighting the initial scrubber failure in cold soaked canisters, followed by an initial effectiveness in absorbing carbon dioxide, followed minutes later by complete scrubber failure. This highlights the importance of managing cold soaked canisters effectively, including pre-breathing protocols. In Antarctica, we used a 5-minute pre-breath protocol, with no noticeable failure in canister efficacy once the dives began, while diving in 28.6oF/-1.8oC water.
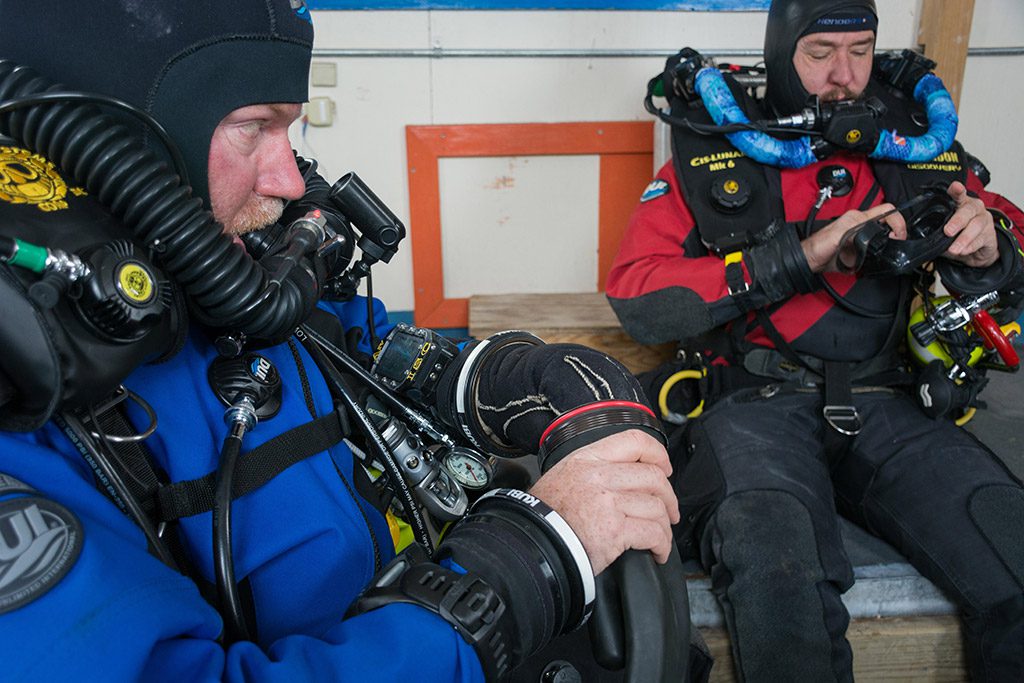
The section on Absorbent Granule Size Distributions (Pages 124-130) returned to a theme introduced on Page 1, variability in absorbent as provided by the manufacturer. You do not always get what you think you are getting. Two other points struck me as particularly important here. The first dealt with friability, or the tendency of absorbent granules to break into smaller particles. I have personally found this to be problematic operationally with some absorbents, including Draeger’s DiveSorb. The other point made in this section was the use by some recreational rebreather divers of absorbents other than those recommended by the manufacturers. This issue has been a long-held concern of mine. In this discussion, John pointedly discussed the use of Spherasorb (a medical absorbent used in anesthesia machines) in place of Molecular Products’ Sofnolime, the manufacturer recommended absorbent for use in their rebreathers (Page 128). Experimentation by Duke and New Zealand researchers showed that time to breakthrough using medical grade Spherasorb was significantly less than when using Sofnolime.
The section labeled Pre-Dive Decision Making is especially important to rebreather users (beginning Page 133). The variables discussed in this section have a direct bearing on real world diving decisions. Topics covered include selection of a fine grain versus a larger grain absorbent, breathing resistance, pressure drop across an absorbent bed, breathing resistance limits, and other physiologic considerations in rebreather use. I will just say to the divers in the audience… “Read it!”
As I read the discussion on gas density and physiologic interactions, I was left with a single question: “Why am I still alive?” My deepest dive depth on air was 86 mfw/280 ffw (not planned—chasing another cave diver who was impaired from narcosis, and who had exceeded the planned depth of 67 mfw/220 ffw). I consciously avoided any work at all, relying on overweighting to bring me down and lift from my BC to bring me up again. This section highlighted that I have been extremely lucky in some of my past dives.
On Page 149, John repeats a question posed to him, “Which is more lethal for rebreather divers, breathing resistance or CO2?” His response: “It’s both!” This statement pointed out the fact that, as rebreather divers, we cannot focus on any single aspect of threat or danger while using this equipment. To focus on one to the exclusion of others engenders risk that may be excessive. We have to focus on multiple variables and manage multiple variables, continually, while diving.
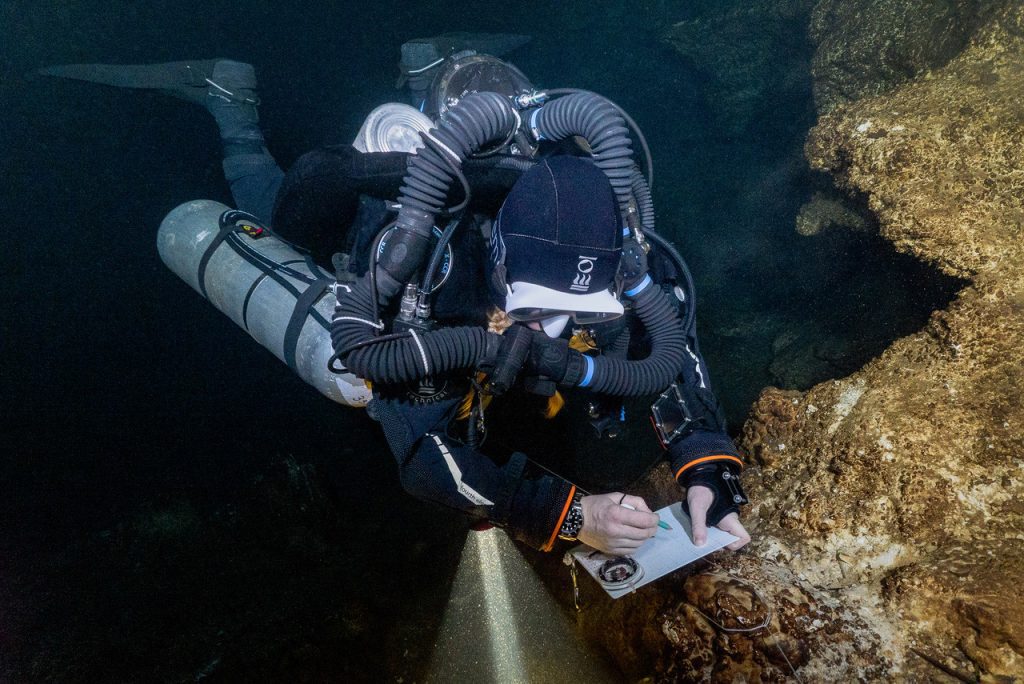
In Conclusion
Conclusions are presented in Chapter 8. This is a concise summary of the major findings in the book. While “skipping to the end of the book” to read these findings gets some of the major concepts across and may give you a feel for some of the material presented, it is not a substitute for reading the manuscript. The discussions, developmental arguments, and conclusions placed in the framework of the modeling and experimentation are well worth reading.
The remainder of the book is a series of Appendices that provide additional supportive data and information, including additional advanced math. John has promised to provide an even deeper review of some of the material in the form of an eBook Supplement that should soon be available and linked to on his website.
These were the sections that I found of interest, but probably because they were based on my personal experiences. I have no doubt that different readers will find other points John makes that engage them more than they do me. There is a wealth of information here, and it is difficult to grasp it all in a single reading.
Overall, I feel this is an excellent book with well-considered points based on experimental data, as well as modeling. It is a valuable addition to any rebreather user’s library.
Dive Deeper
InDEPTH: Too Much to Absorb: What You Need to Know About Your Scrubber by Reilly Fogarty (2020)
InDEPTH: Estimating Your Scrubber Duration
InDEPTH: InDepth’s Holiday Rebreather Guide: 2022 Update
InDEPTH: How Deep Is Your Library?
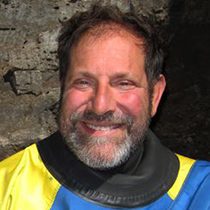
Jeffrey Bozanic PhD is a technical diving instructor and research scientist. Based in southern California, Jeff provides consulting and training services in the diving market. Specializing in rebreather use, he is probably best known for his seminal textbook on the topic, Mastering Rebreathers, and his work as senior Technical Editor for the 6th Edition of the NOAA Diving Manual.
A marine scientist, Jeff has participated or lead over 60 diving expeditions during the past 40 years, to places like Palau, Africa, Tonga, and Mexico. From 1989-1992 he oversaw scientific diving operations in Antarctica for the U.S. Antarctic Program, including co-authoring the Antarctic Scientific Diving Manual. In 2016 he was part of a research team that tested and evaluated rebreathers in Antarctica for the National Science Foundation.
Jeff has served on many Boards, including as NSS-CDS Chairman, NAUI Vice Chairman, and AAUS Treasurer. He has been honored with the NAUI Lifetime Achievement Award, DAN/Rolex Diver of the Year, and AAUS Conrad Limbaugh Award for Scientific Diving Leadership.